The last two decades have witnessed a rapid increase in the number of inherited disorders caused by ion channel mutations. One of the major challenges is to delineate the full clinical spectrum of these ‘channelopathies’. Among this expanding group, neurological ion channel disorders are among the best characterised, reflecting the fundamental importance of electrical excitability in the membranes of nerve and muscle cells. The neurological ion channelopathies may be divided into those that affect skeletal muscle and those that affect neurons. A common feature is that they often manifest clinically as paroxysmal attacks of paralysis, myotonia, or brain, spinal cord and nerve dysfunction. This review focuses on CNS channelopathies with the aim of providing an overview of recent advances and identifying gaps in our knowledge of disease mechanisms.
Introduction
Neurological ion channel diseases may be broadly classified as ‘acquired’or ‘genetic’. Acquired channelopathies are generally caused by antibodies that target specific ion channels or by toxins and venoms which block voltage-gated ion channels. Altered transcription, assembly, and membrane trafficking of ion channels may also contribute to many acquired neurological ion channel disorders. The acquired channelopathies are not the subject of this article. Instead, this review is focused on the genetic neuronal ion channel disorders and specifically, those inherited or de novo mutations in ion channel subunits that result in brain dysfunction. The genetic skeletal muscle channelopathies, which lie at the other end of the anatomical spectrum and which are characterised by myotonia or periodic paralysis have been comprehensively reviewed elsewhere.1
A striking feature of most of the known CNS channelopathies is that they manifest as paroxysms of disturbed function against a background of normal development. Genetic ion channel disorders of the brain generally manifest as epilepsy, migraine, paroxysmal dyskinesia, or episodic ataxia. Although individually rare, the expectation is that the study of these monogenic disorders will help to illuminate the pathophysiological basis of paroxysmal disorders in general and in particular the mechanistic pathways involved in common forms of epilepsy and migraine. Whilst a comprehensive review of the brain ion channel disorders is beyond the ambition of this article, our aim is to provide a brief overview of the field and consider the most recent advances, especially from a mechanistic point of view.
Brain ion channels and disease
Epilepsy
Although the genetic basis of idiopathic generalised epilepsy (IGE) remains a mystery, our understanding of the Mendelian or monogenic epilepsies has in contrast progressed considerably. Mutations in genes which encode subunits of CNS sodium, potassium, calcium channels, GABAA and nicotinic receptors have been reported in association with various epilepsy syndromes2-3 (Table 1).
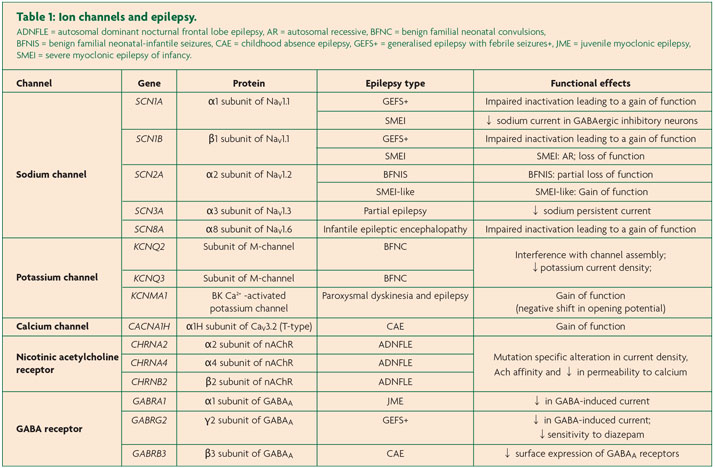
Benign familial neonatal convulsions (BFNC) is a disorder characterised by frequent brief attacks of partial and generalised seizures which typically resolve by six weeks of age. It was the first epilepsy disorder for which gene linkage studies established a disease locus, and subsequently identified a pair of potassium channel genes that had not previously been cloned. Mutations in both KCNQ2 and to a lesser extent in KCNQ3 have been reported with BFNC.2 Some cases of severe epileptic encephalopathy have recently been linked to mutations of KCNQ2. Other potassium channel genes implicated in epilepsy include KCNMA1 in which a dominant missense change was identified in a single large family with paroxysmal dyskinesia and epilepsy,4 and KCNA1, the gene mutated in episodic ataxia type 1 (EA1 – see below) which not uncommonly is complicated by seizures.5 Generalised epilepsy with febrile seizures plus (GEFS+) is a clinically and genetically heterogeneous epilepsy disorder which manifests in childhood as febrile seizures which may be atypical (hence ‘+’), afebrile generalised seizures, and in rare cases myoclonic astatic epilepsy. Kindreds with GEFS+ show dominant inheritance but different affected members can present with strikingly different seizure types and severities. Although, the first disease-causing mutation was identified in SCN1B, which encodes the β1 auxiliary subunit of the voltage-gated sodium channel,6 mutations in SCN1A, which encodes the pore-forming α subunit of the neuronal sodium channel NaV1.1, have since been identified.7 GEFS+ can also be caused by heterozygous missense mutations in GABRG2 which encodes the γ2 subunit of the GABAA receptor, which mediates fast inhibitory neurotransmission in the CNS. The mechanisms underlying epilepsy associated with γ2 subunit mutations remain unclear.
Mutations in the SCN1A gene may also lead to severe myoclonic epilepsy of infancy (SMEI or Dravet syndrome).2 Affected individuals present in the first year of life with prolonged clonic or tonic-clonic seizures associated with febrile illness, and subsequently develop other seizure types including myoclonic and absence seizures. In addition, speech and motor development is impaired and ataxia occurs. Seizures often respond poorly to antiepileptic drugs. Approximately 70% of patients with SMEI have missense or nonsense mutations of SCNA1, and several hundred different mutations have now been identified. These tend to be de novo mutations leading to haploinsufficiency. That loss of function of a sodium channel gene should lead to epilepsy is paradoxical. A novel insight into disease mechanisms in this disorder came from a mouse model of SMEI which revealed a reduction in sodium current density in inhibitory interneurons, resulting in circuit disinhibition.8 This model also accounts for worsening of seizures with anti-epileptic drugs that target sodium channels. SMEI has also been reported, albeit rarely, in association with mutations in SCN1B, which encodes the β1 subunit of the voltage-gated sodium channel9 and SCN2A, which encodes the α2 subunit of NaV1.2.10. Missense mutations in SCN2A are also associated with benign familial neonatal-infantile seizures (BFNIS) in a few families. BFNIS is characterised by tonic or clonic partial and generalised seizures with onset between 2 days and 3.5 months. Recently, a gain of function mutation in SCN8A, which encodes NaV1.6, was identified in a family affected by infantile epileptic encephalopathy.11
Autosomal dominant nocturnal frontal lobe epilepsy (ADNFLE) is a rare disorder characterised by frequent short-lived seizures during sleep with a prominent motor component, usually consisting of violent movements and dystonic posturing of the limbs, often with preserved consciousness. Mutations in CHRNA4, CHRNA2 and CHRNB2 which encode the α4, α2 and β2 subunits of the neuronal nicotinic acetylcholine receptor (nAChR) respectively are associated with ADNFLE. The mechanisms linking altered nAChR function to focal neocortical seizures remain poorly understood.
Heterozygous mutations in GABRB3, which encodes the β3 subunit of GABAA are associated with childhood absence epilepsy, and one loss of function mutation in GABRA1, which encodes the α-subunit of the GABAA was identified in a large family with juvenile myoclonic epilepsy.12
Finally, genetic defects, including rare variants in calcium channel genes such as CACNA1H, which encodes the α1H subunit of a T-type calcium channel (CaV3.2) which is highly expressed in the thalamus, and in CACNA1A (see below and Tables 2 and 3), have been associated with childhood absence epilepsy13 and EA2 with epilepsy respectively.14 However these tend not to co-segregate with epilepsy and so they may be considered susceptibility factors.
Ataxia
Ion channel mutations cause both episodic and progressive forms of ataxia. With regard to the former, dominantly inherited episodic ataxia types 1 (EA1) and 2 (EA2) are the most common types15 (Table 2). EA3 – EA8 are rare, having generally only been reported in single families, and will not be discussed further.
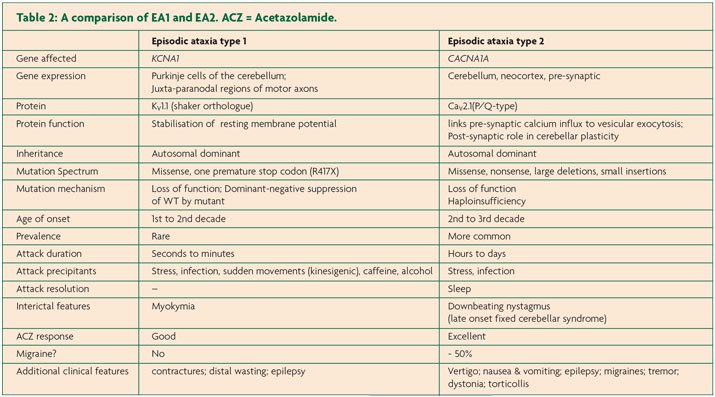
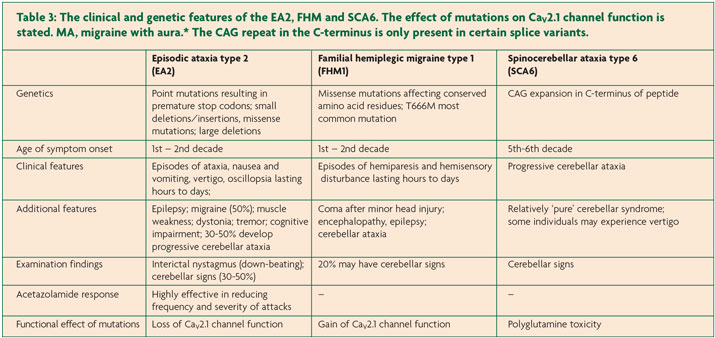
Attacks in EA1 are short-lived (seconds to minutes) and affected individuals are normal interictally, although may exhibit neuromyotonia – a consequence of peripheral nerve hyperexcitability. In contrast, the attacks in EA2 are more severe, and can last from hours to days and may be associated with vertigo, vomiting and oscillopsia. The interictal examination may reveal deficits in oculomotor function, including gaze-evoked nystagmus. In addition, approximately 30-50% of genetically confirmed EA2 cases develop a progressive cerebellar syndrome.
EA1 is associated with heterozygous missense changes in KCNA1, although a premature stop codon has been identified in one family. KCNA1 encodes Kv1.1, a potassium channel subunit that occurs throughout the nervous system but is especially abundant in the terminals of inhibitory interneurons in the cerebellar cortex, as well as in motor axons. Channels containing Kv1.1 are thought to regulate axonal excitability and neurotransmitter release, and mutant subunits generally exert a dominant-negative effect on wild type subunits with which they co-assemble. Why EA1 is paroxysmal is not known.
A wide range of heterozygous mutations in CACNA1A have been identified in EA2, ranging from missense substitutions to splice site mutations and stop codons that are predicted to truncate the peptide. Large scale deletions of multiple exons have recently also been identified to cause EA2 in patients previously reported as ‘CACNA1A-negative’.16 The gene encodes the α1A subunit of the Cav2.1 calcium channel, also known as the P/Q-type channel. This channel is abundantly expressed at most presynaptic terminals throughout the nervous system, where it plays an important role in triggering neurotransmitter release. It is also expressed at high levels in the dendrites and somata of cerebellar Purkinje and granule cells.
Mutations in CACNA1A also underlie spinocerebellar ataxia type 6 (SCA6), a relatively ‘pure’ progressive degenerative disease of the cerebellum without paroxysmal features. This is caused by an expansion of a polyglutamine (CAG) repeat in the intracellular C-terminus of the α1Achannel peptide, although missense mutations have also been identified.17 A toxic gain of function has been suggested to underlie the disease mechanism, akin to that observed in Huntington’s disease, but the evidence is indirect. The mechanism of cell death induced by the polyQ tract remains unclear although it has been suggested that cerebellar dysfunction in a related disorder, SCA2, may arise from aberrant activation of type 1 inositol 1,4,5-trisphosphate receptors (IPTRs) in Purkinje cells by the glutamine tracts themselves.18 More recently, heterozygous mutations in KCNC3, which encodes the potassium channel, KV3.2, have been identified in association with early and adult-onset progressive ataxia and neurodevelopmental delay, termed SCA13. The pathological mechanism is unclear.19
Migraine
Heterozygous missense mutations in CACNA1A underlie Familial Hemiplegic migraine type 1 (FHM 1), a severe subtype of migraine with aura causing disabling attacks of hemiplegia, paraesthesia and visual disturbance which may last from hours to days. Additional features rarely include delayed cerebral oedema and coma after a minor head injury, confusion, encephalopathy, and a cerebellar syndrome in approximately 20% of cases. Mechanistically, the mutations are thought to result in a gain of function, with a knock-in mouse model of FHM1 exhibiting enhanced spontaneous transmission at the neuromuscular junction and a lower threshold for inducing cortical spreading depression (CSD), the physiological substrate for the aura of migraine.20 Genetic heterogeneity is also a feature of FHM. Apart from CACNA1A, two other genes have been associated with Mendelian hemiplegic migraine: FHM2 is caused by loss-of-function mutations in ATP1A2, which encodes the alpha subunit of a sodium/potassium ATPase which helps maintain transmembrane ion gradients. FHM3 is caused by mutations in the neuronal sodium channel gene, SCN1A, which is also affected in GEFS+ and SMEI (see above). It is not clear why some SCN1A mutations lead to migraine rather than epilepsy.
Hyperekplexia
Familial Hyperekplexia (FH) was the first CNS ion channel disorder in which a mutation was identified.21 It is characterised clinically by stiffness and an exaggerated startle reaction to sensory or auditory stimuli, which is thought to have its origin in the brainstem reticular formation. The disorder presents in infancy and can result in life-threatening apnoeic spells, although treatment with benzodiazepines is helpful and attacks become less marked with age. FH generally arises from missense mutations in the GLRA1 gene, which encodes the α1 subunit of the glycine receptor that contributes to fast inhibition in the brainstem and spinal cord. Impairment of inhibition is thought to result in excessive motor activity, explaining the exaggerated startle response and co-contraction of muscles. Although most cases are autosomal dominant, sporadic cases and recessive mutations have also been described. Compound heterozygous mutations in GLRB, which encodes the β-subunit of the glycine receptor, have been identified in association with a few cases of hyperekplexia, as have mutations of the glycine transporter GlyT2.
Conclusion
Although individually rare, the channelopathies are of growing importance in neurological disease, not least because of the key insights they provide about the function of ion channels, their roles in membrane excitability and their interactions with various signalling cascades. Such knowledge will not only lead to a greater understanding of the basis of common paroxysmal disorders but may in time provide important therapeutic avenues. Furthermore, because of the direct evidence that their dysfunction gives rise to migraine, epilepsy and movement disorders, ion channel genes are the best candidate loci for polygenic inheritance of common forms of these diseases. However, many challenges lie ahead. From a mechanistic point of view, whilst electrophysiological techniques have demonstrated changes in the biophysical parameters of mutant channels, how these alterations lead to paroxysmal or progressive symptoms is largely unknown. Indeed why symptoms are classically paroxysmal in the first place when the genetic lesion is constant is still unclear, as are the reasons why certain disorders such as BNFC have an early onset and run a benign course whereas others such as SMEI or KCNC3-related channelopathies exhibit a more severe and progressive phenotype.
References
- Cannon SC. Pathomechanisms in channelopathies of skeletal muscle and brain. Annu Rev Neurosci, 2006;29:387-415.
- Gourfinkel-An I, et al. Monogenic idiopathic epilepsies. Lancet Neurol, 2004;3(4):209-18.
- Kullmann DM. Neurological channelopathies. Annu Rev Neurosci, 2010;33:151-72.
- Du W, et al. Calcium-sensitive potassium channelopathy in human epilepsy and paroxysmal movement disorder. Nat Genet, 2005;37(7):733-8.
- Rajakulendran S, et al. Episodic ataxia type 1: a neuronal potassium channelopathy. Neurotherapeutics, 2007;4(2):258-66.
- Wallace RH, et al. Febrile seizures and generalized epilepsy associated with a mutation in the Na+-channel beta1 subunit gene SCN1B. Nat Genet, 1998;19(4):366-70.
- Escayg A, et al. Mutations of SCN1A, encoding a neuronal sodium channel, in two families with GEFS+2. Nat Genet, 2000;24(4):343-5.
- Yu FH, et al. Reduced sodium current in GABAergic interneurons in a mouse model of severe myoclonic epilepsy in infancy. Nat Neurosci, 2006;9(9):1142-9.
- Patino GA, et al. A functional null mutation of SCN1B in a patient with Dravet syndrome. J Neurosci, 2009;29(34):10764-78.
- Shi X, et al. Clinical spectrum of SCN2A mutations. Brain Dev, 2012;34(7):541-5.
- Veeramah KR, et al. De novo pathogenic SCN8A mutation identified by whole-genome sequencing of a family quartet affected by infantile epileptic encephalopathy and SUDEP. Am J Hum Genet, 2012;90(3):502-10.
- Cossette P, et al. Mutation of GABRA1 in an autosomal dominant form of juvenile myoclonic epilepsy. Nat Genet, 2002;31(2):184-9.
- Khosravani H and Zamponi GW, Voltage-gated calcium channels and idiopathic generalized epilepsies. Physiol Rev, 2006;86(3):941-66.
- Rajakulendran S, et al. Genetic and functional characterisation of the P/Q calcium channel in episodic ataxia with epilepsy. J Physiol, 2010;588(Pt 11):1905-13.
- Jen JC, et al. Primary episodic ataxias: diagnosis, pathogenesis and treatment. Brain, 2007;130(Pt 10):2484-93.
- Labrum RW, et al. Large scale calcium channel gene rearrangements in episodic ataxia and hemiplegic migraine: implications for diagnostic testing. J Med Genet, 2009;46(11):786-91.
- Zhuchenko, O., et al., Autosomal dominant cerebellar ataxia (SCA6) associated with small polyglutamine expansions in the alpha 1A-voltage-dependent calcium channel. Nat Genet, 1997;15(1):62-9. \
- Liu J, et al. Deranged calcium signaling and neurodegeneration in spinocerebellar ataxia type 2. J Neurosci, 2009;29(29):9148-62.
- Waters MF, et al. Mutations in voltage-gated potassium channel KCNC3 cause degenerative and developmental central nervous system phenotypes. Nat Genet, 2006;38(4):447-51.
- van den Maagdenberg AM, et al. A Cacna1a knockin migraine mouse model with increased susceptibility to cortical spreading depression. Neuron, 2004;41(5):701-10.
- Shiang R, et al. Mutations in the alpha 1 subunit of the inhibitory glycine receptor cause the dominant neurologic disorder, hyperekplexia. Nat Genet, 1993;5(4):351-8.