Abstract
The non-invasive exploration of the peripheral nervous system using magnetic resonance imaging (MRI) has recently gained momentum. The use of basic and advanced MRI protocols has allowed for both qualitative and quantitative assessment of the peripheral nerves (PN), enabling the structural and functional changes of peripheral pathology to be increasingly investigated. From a clinical perspective, this has impacted diagnosis, treatment and monitoring across a variety of conditions. This review will provide an overview of the current MRI protocols used for PN evaluation, the application of this in the clinical setting, and the expanding techniques within the research field.
Introduction
Peripheral nerves (PN) are involved in a myriad of pathologies and represent a significant burden of disease. Gold standard evaluation currently relies on both clinical and electrodiagnostic testing, but this bestows certain challenges, particularly in early stages of disease when signs and symptoms may be insidious.1 As such, neuromuscular medicine is not uncommonly marred by diagnostic uncertainty, complicating management and treatment decisions.
Although magnetic resonance imaging (MRI) commands a pivotal role in the clinical assessment of neurological disorders, early analysis focused on the central nervous system leaving peripheral nerve techniques relatively unsophisticated. Since MR visualisation of the peripheral nerve was eventually pioneered in 1992,2 developments in this field have now accelerated this tool into the clinical spotlight.3 This review provides an overview of the MRI techniques to assess peripheral nerves, and will outline current clinical applications. Emerging research in this field will also be summarised.
Peripheral nerve MR techniques
Peripheral nerves can now be directly visualised using basic and advanced MRI protocols, allowing differentiation of various pathologies at a structural and functional level (Table 1).
Conventional MRI
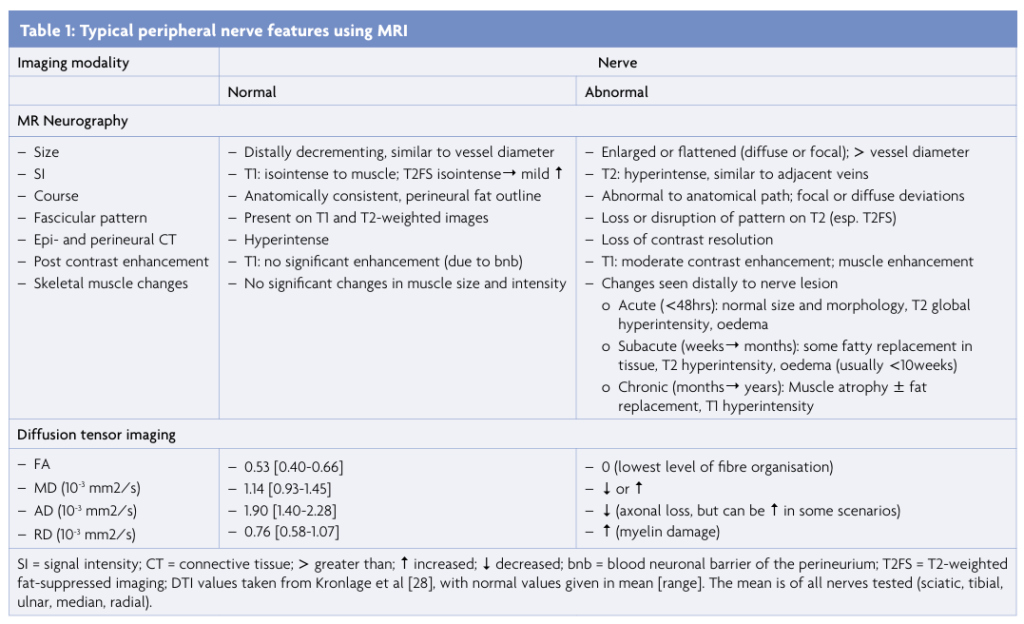
MRI provides important information about the structural integrity of the nerve. Understanding the microstructural organisation of this pathway is a crucial step for accurate interpretation of imaging findings (Figure 1).4 Briefly, the axon is encased by the endoneurium and surrounded by endoneurial fluid. This fluid is a main determinant of signal characteristics on non-contrast T2 scans, appearing moderately hyperintense in the absence of pathology. The nerve fascicle is the smallest unit that can be visualised on MRI, insheathed by the perineurium. This layer acts as a blood-nerve-barrier, and thus no gadolinium contrast enhancement should be seen in healthy nerves.1,3
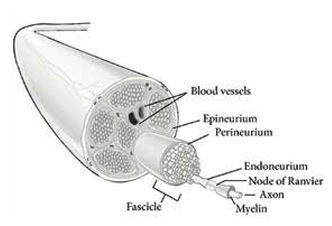
Figure 1: Peripheral nerve anatomy. Schematic drawing of a large peripheral nerve. The epineurium insheathes a collection of fascicles interspersed with adipose tissue. Each fascicle (enlarged) is surrounded by a perineurium and contains axons (myelinated and unmyelinated) that are each enclosed by endoneurial connective tissue. Adapted from [4].
MR neurography (MRN) is a specifically designed PN protocol that delivers a higher level of anatomic detail. This usually incorporates a T1-weighted image that highlights the fascicular ultrastructure of the nerve, often combined with a T2-weighted image that is modified to suppress non-neural structures (e.g. fat and vasculature). The latter sequence enhances water-based pathology, such as oedema and nerve inflammation.3 High resolution phased-array coils can also be used to increase anatomical detail, making fascicles appear more hyperintense and nerve characteristics (including calibre, course and size) easier to identify.3
Advanced MRI techniques
Quantitative assessment of PN pathology is possible through advanced MRI sequences, providing valuable pathophysiological information regarding the functional integrity of the nerve. Currently, techniques for the PN are largely based on diffusion imaging, which measures a wide range of nerve properties.5
Diffusion imaging
MR diffusion imaging is based on the concept that water molecules are highly directional (‘anisotropic’), effectively restricted to move along the linear nerve pathways, hindered by intact cell membranes and macromolecules (Figure 2A).5-7 Any damage to this microstructural integrity results in less restriction of water diffusion along this path and a loss of this directionality (Figure 2B).6 MR diffusion tensor imaging (DTI) provides metrics to interpret directionality, creating a numerical approach to evaluate nerve integrity. Peripheral DTI correlates with neurophysiological and histological nerve changes in a number of studies,5,7,8 and the reliability and reproducibility of this technique in healthy peripheral nerves was recently established.5
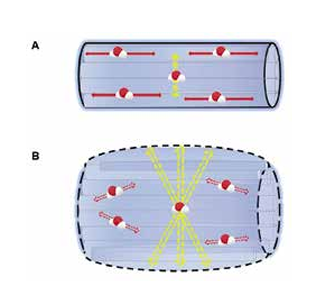
Figure 2: Principles of DTI. A. In a normal PN with preserved axonal integrity, water molecules diffuse along one main direction (red arrows) and have low perpendicular movement (yellow arrow) due to the physiological barriers of the intact nerve. B. An injured PN demonstrates a loss of fibre organisation (dashed black lines), with subsequent loss of directionality of water molecule movement (red arrows). Loss of perpendicular barrier integrity (i.e. myelin sheath) increases movement in this direction (yellow arrows).
The common DTI parameters are (Figure 2):
(i) fractional anisotropy (FA); indicating how strongly ‘directional’ water diffusion is within the tissue, inferred to reflect the structural integrity of the pathway;
(ii) mean diffusivity (MD); reflecting water displacement distance independent of direction;
(iii) axial diffusivity (AD); representing diffusion in parallel (axoplasmic) to the nerve tract. AD is most specific for axonal integrity, and has been reported as an indicator of axonal damage;9
(iv) radial diffusivity (RD); representing diffusion in a perpendicular direction to the nerve tract. RD probably reflects myelin integrity and has been related to changes in myelin pathology.9
These DTI datasets can also be reconstructed to give a 3D representation of nerve fibre orientation using diffusion tensor tractography (DTT) (Figure 3).7,10
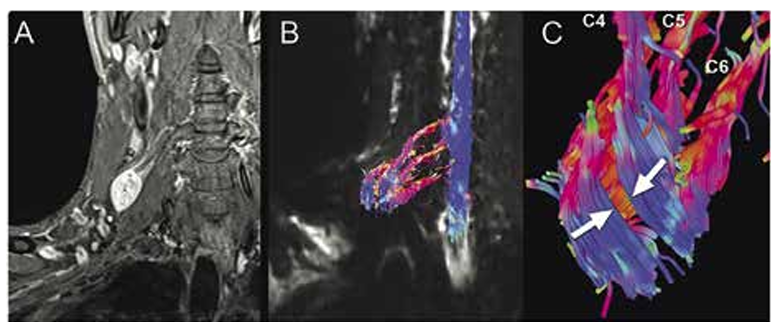
Figure 3: 3D nerve reconstruction using DTT.
3D neurography using diffusion tensor tractography (DTT) to visualise nerve fibres surrounding a brachial plexus tumour. A. Postcontrast T1 image of an enhancing right C4 schwannoma. B,C. Tractography images demonstrate the nerve fibres surrounding the tumour. The white arrows (C) show thinning along the anterior face. Reproduced with permission [10].
Clinical application of peripheral nerve MRI
Although clinical and electrodiagnostic testing remain at the core of PN evaluation and diagnosis, false negative results can be common, particularly during the early phase of disease, in very proximal nerves, or when nerve injury is mild.11 Neurophysiological assessment can also be invasive and uncomfortable, and may be impacted by technical issues and heterogenous disease pathology. Such factors can lead to a failure to localise pathology to a specific nerve segment and may hinder treatment decisions.
MRI is a particularly useful tool in these settings, with techniques increasingly applied to a wide range of PN pathologies including traumatic nerve injuries, non-traumatic neuropathies (such as inherited and immune-mediated disorders) and nerve tumours.7,12-14 Practically, PN imaging is usually performed on three Tesla (3T) MRI field strength scanners. Standard protocols (such as MRN) can be performed with basic MRI facilities, while DTI protocols often require technical expertise. The growing use of MRI in practice is allowing crucial clinical questions to be more accurately addressed, such as (i) localisation of disease pathology, (ii) measurement of injury severity and (iii) monitoring of nerve recovery.
(i) Localising pathology
Basic MRI can localise structural pathology, such as focal nerve swelling at sites of conduction block in inflammatory polyneuropathies.15 Locating the lesion in carpal tunnel syndrome (CTS), the most common form of entrapment neuropathy (caused by median nerve compression at the wrist), may also require MRI when limited by normal electrophysiological tests in early disease.11,16 In this clinical setting, T2-hyperintense signal changes are seen 24 hours post-injury at (and distal to) the site of pathology. Three main signs subsequently evolve on imaging to confirm entrapment neuropathy: (i) intraneural oedema, (ii) nerve enlargement, and (iii) gadolinium enhancement (in some instances). MRI can also explore the specific level and aetiology of compression, indirectly aided by the presence and distribution of muscle denervation around the nerve(s) involved (Table 1).16
The location of PN tumours involving the nerve sheath can also be identified on basic MRI by way of a ‘fat-split’ sign, which occurs around the intermuscular space or neurovascular bundle. Characteristic MRI features have also been described to differentiate between malignant and benign nerve tumour forms, such as a large size and perilesional oedema (causing indistinct margins and peripheral enhancement) in the case of the former.17
(ii) Measuring disease severity
Identifying the severity of nerve injury is essential to determine the most efficacious treatment and the likelihood of recovery.7 Nerve injury grading systems, established by Seddon and adapted by Sunderland (Table 2),18,19 represent a continuum ranging from mild damage involving focal nerve demyelination with no axonal involvement (neuropraxia/stage I), to complete nerve tears with a focal loss of axons (neurotmesis/stage V) (Table 2). Although electrophysiology remains the mainstay of such assessment, these changes can be challenging to resolve in the first six weeks and MRI may be of additional benefit by identifying early disruption to nerve ultrastructure. MRI can also facilitate distinction between the very severe levels of injury that are identical on electrophysiological testing, as in the case of axonotmesis (i.e. focal loss of axons with preservation of nerve connective tissue) versus neurotmesis (i.e. focal loss of axons and nerve connective tissue elements) (Table 2). Although axonotmesis may recover spontaneously, neurotmesis requires early surgical intervention. As such, clinical distinction is paramount in order to guide treatment.20
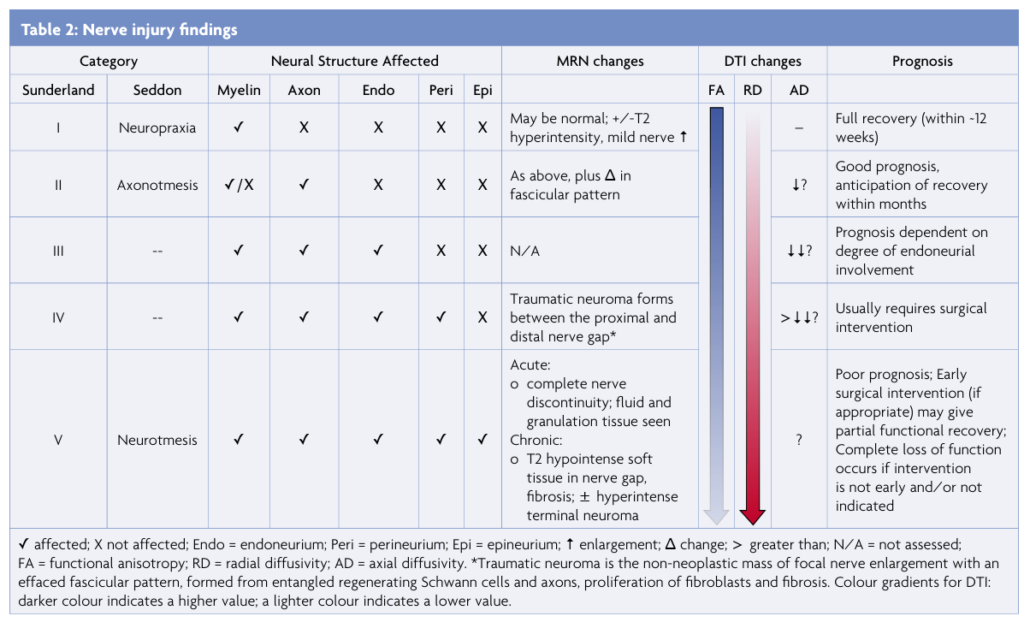
(iii) Monitoring nerve recovery
MR modalities can, in theory, also offer a non-invasive alternative for routine monitoring of nerve recovery post-injury, particularly when repeating electrophysiology may not be practical. In some case, early normalisation of MRN changes has been reported prior to neurophysiological evidence of recovery, and may be useful in relapsing inflammatory conditions or if infiltrative lesions are of concern.15 MRI measurement of axonal degeneration has also been reported in conditions such as amyotrophic lateral sclerosis (ALS), a rare and progressive terminal disorder, with correlation between disease progression and increased brachial plexus signal on T2-weighted sequences described.21
Ultimately however, these dynamic nerve changes can take months to resolve on MRN, and as such this has not been adopted for routine use in clinical practice.
Future developments
The growth of MRI technology continues to promote experimental techniques for PN imaging, which are currently being developed within the research spectrum. With each technique offering their own strengths and weakness (Table 3), the combined use of quantitative and qualitative assessments is particularly beneficial, providing unique clinical advantages to PN characterisation that include insights into the dynamics of nerve injury and regeneration.
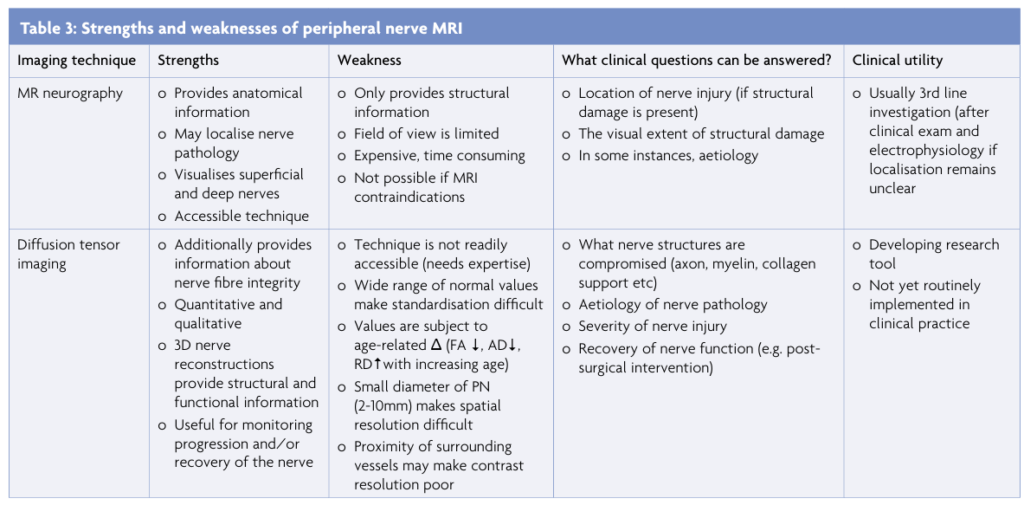
7T MRI
7T MRI imaging of the PN has only recently been attempted in a small pilot study involving three healthy volunteers.22 This demonstrated much clearer visualisation of nerve fascicules in comparison to 3T imaging (Figure 4A-D). Although feasible, the availability of 7T scanners, a longer scan time, potentially increased sensitivity to side-effects, and the need for different receiver coils have limited the immediate use of this protocol in the clinical setting.
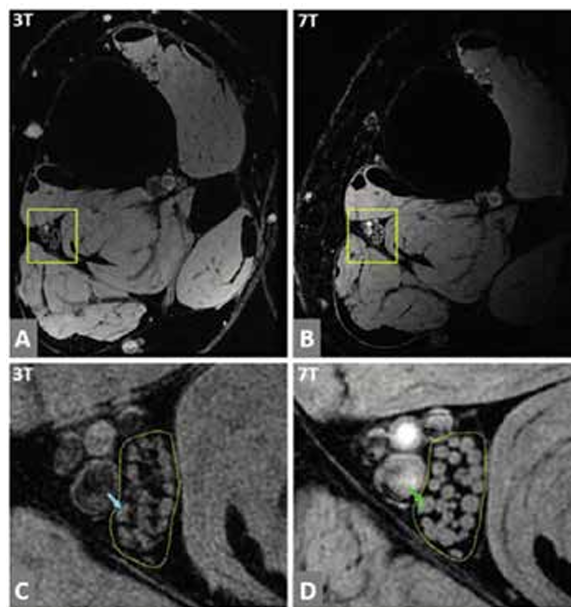
Figure 4: Peripheral nerve imaging using 7T MRI.
Tibial nerve fascicles are depicted on high resolution images acquired at 3T and 7T. A and B show full images at 3T and 7T, respectively. C. Enlarged version within the outlined yellow box in A, showing tibial nerve fascicles in detail at 3T. D. Enlarged version within the outlined yellow box in B, showing the same nerve image at 7T. The yellow dotted line (C,D) outlines the tibial nerve fascicles, and a single fascicle is seen marked by the linear line in C and D (as highlighted by the blue and green arrow, respectively). Overall, the fascicular structure has a clearer definition on 7T (D). Reproduced with permission [22].
DTI
DTI can specify pathophysiological changes affecting nerve fibre integrity (such as loss of myelin, presence of oedema, or axonal loss), which is particularly useful in circumstances where structural changes are subtle and difficult to identify using standard MRI. In CTS, a reduction of FA correlates with electrodiagnostic studies and has been reported to hold a diagnostic sensitivity and specificity of 82.2% and 77.8%, respectively.23 Non-invasive localisation and differentiation between benign and malignant nerve tumours may also be facilitated with DTI, with a relatively lower FA and higher MD seen in cases of malignancy.13 3D nerve reconstructions with DTT have been particularly useful for pre-operative planning in this regard, depicting the relationship of the tumour to the remaining nerve fibre (with a mapping sensitivity of 95.7% and specificity of 66.7%). The use of this technique has resulted in a changed surgical strategy in 25% of reported cases.24
The dynamic change of DTI parameters (particularly FA) during the functional stages of recovery has been demonstrated as a marker of PN regeneration and an indicator of treatment efficacy.8,25 This has mainly been evaluated after traumatic nerve injury, with a gradual increase of FA occurring between six weeks to six months in patients who show clinical improvement.12,20 3D nerve reconstruction has also had a role in the post-injury phase and can visualise axonal regeneration.7,12 These findings correlate with electrophysiological studies and probably reflect PN axonal growth and regeneration, restoring the nerve towards a normal (anisotropic) structure. Equally, axonal degeneration can be identified by a gradual decline in FA and AD metrics in the lower limb of ALS patients, demonstrated over a six-month period.14 In such conditions where robust biomarkers are truly lacking, these parameters may offer an important indication of disease prognosis.
Conclusion
The use of non-invasive, novel MRI techniques has enhanced understanding of the structural and functional architecture of PN pathology. The evolution of these techniques for the PN has provided multifaceted information to pilot diagnosis and clinical decision making and has allowed objective monitoring of treatment efficacy. A consensus between the protean MRI protocols are now well poised to be validated in larger cohorts, which will hopefully verify robustness and repeatability of technique. This will be essential for successful translation into the routine clinical and diagnostic rhetoric of PN assessment, and for reliable interpretation of pathology. Ultimately, the current exponential growth of peripheral MRI techniques may soon enable dynamic disease characterisation at an individual patient level, in-line with a modern era of personalised medicine.
References
- Zaidman C, Seelig M, Baker J, Mackinnon S, Pestronk A. Detection of Peripheral Nerve Pathology. Neurology 2013, 80:1634-1640.
- Howe F, Filler A, Bell B, Griffiths J. Magnetic resonance neurography. Lancet 1992; 341(8846):659-661.
- Simon N, Talbott J, Chin C, Kliot M. Peripheral Nerve Imaging. Handbook of Clinical Neurology. 2016;136:811-826.
- Grinsell D, Keating C. Peripheral Nerve Reconstruction after Injury: A Review of Clinical and Experimental Therapies. BioMed Research International. 2014;2014:1-13.
- Simon N, Lagopoulos J, Gallagher T, Kliot M, Kiernan M. Peripheral nerve diffusion tensor imaging is reliable and reproducible. J Magn Reson Imaging. 2016;43:962-969.
- Beaulieu C. The basis of anisotropic water diffusion in the nervous system – a technical review. NMR Biomed. 2002;15:435-455.
- Noguerol T, Barousse R, Socolovsky MAL. Quantitative magnetic resonance (MR) neurography for evaluation of peripheral nerves and plexus injuries. Quant Imaging Med Surg. 2017;7(4):398-421.
- Simon N, Kliot M. Visualising axonal degeneration and regeneration with diffusion weighted MRI. Neural Regen Res. 2014;9(24):2122-2124.
- Wheeler-Kingshott C, Cercignani M. About “axial” and “radial” diffusivities. Magn Reson Med. 2009;61:1255-1260.
- Gallagher T, Simon N, Kliot M. Vizualising nerve fibers surrounding a brachial plexus tumour using MR diffusion tensor imaging. Neurology. 2016;86(6):582-583.
- Kimura J. Electrodiagnosis in diseases of nerve and muscle: principles and practice, 4th edition edn: Oxford University Press. 2013.
- Simon N, Narvid J, Cage T, Banerjee S, Ralph J, Engstrom J, Kliot M, Chin C. Visualising axonal regeneration after peripheral nerve injury with magnetic resonance tractography. Neurology. 2014;83:1382-1384.
- Cage T, Yu E, Hou S, Birk H, Simon N, Noss R, Rao A, Chin C, Kliot M. Magnetic Resonance Diffusion Tensor Imaging can visualise nerve fibers and their relationship to peripheral nerve tumours. Neurosurg Focus. 2015;39(3).
- Simon N, Lagopoulos J, Paling S, Pfluger C, Park S, Howells J, Gallagher T, Kliot M, Henderson R, Vucic S et al. Peripheral nerve diffusion tensor imaging as a measure of disease progression in ALS. J Neurol. 2017;264(5):882-890.
- Ishikawa T, Asakura K, Mizutani Y, Ueda A, Murate K, Hikichi C, Shima S, Kizawa M, Komori M, Murayama K et al. MR neurography for the evaluation of CIDP. Muscle Nerve. 2017; 55:483-489.
- Bashir W, Connell D. Imaging of entrapment and compressive neuropathies. Semin Musculoskelet Radiol. 2008;12:170-182.
- Wasa J, Nishida Y, Tsukushi S, Shido Y, Sugiura H, Nakashima H, Ishiguro N. MRI features in the differentiation of malignant peripheral nerve sheath tumours and neurofibromas. AJR Am J Roentgenol. 2010;194:1568-1574.
- Seddon H, Medaware P, Smith H. Rate of regeneration of peripehral nerves in man. J Physiol. 1943;102:191-215.
- Sunderland S. A classification of peripehral nerve injuries producing loss of function. Brain. 1951;74:491-516.
- Simon N, Spinner R, Kline D, Kliot M. Advances in the neurological and neurosurgical management of peripheral nerve trauma. JNNP 2015;87:198-208.
- Gerevini S, Agosta F, Riva N, Spinelli E, Pagani E, Caliendo G, Chaabane L, Copetti M, Quattrini A, Comi G et al. MR imaging of brachial plexus and limb-girdle muscles in patients with amyotrophic lateral sclerosis. Radiology. 2016;279:553-561.
- Yoon D, Biswal S, Rutt B, Lutz A, Hargreaves B. Feasibility of 7T MRI for imaging fasciular structures of peripheral nerves. Muscle & Nerve. 2017;57:494-498.
- Lindberg P, Feydy A, Le Viet D, Maier M, Drape J. Diffusion tensor imaging of the median nerve in recurrent carpel tunnel syndrome- initial experience. Eur Radiol. 2013;23:3115-3123.
- Kasprian G, Amann G, Panotopoulos J, Schmidt M, Dominkus M, Trattnig S, Windhagger R, Prayer D, Nobauer-Huhmann I. Peripheral nerve tractography in soft tissue tuomurs: a preliminary 3-Tesla diffusion tensor magnetic resonance. Muscle Nerve. 2015;51:338-345.
- Gallagher T, Simon N, Kliot M. Diffusion tensor imaging to visualize axons in the setting of nerve injury and recovery. Neurosurg Focus. 2015;39:E10.
One comment on “Clinical and research applications of peripheral nerve MRI”