Abstract
Our understanding of the mechanisms underlying coagulation have advanced rapidly in recent years. In addition, there are new methods to assess coagulation and new agents in the treatments of thromboembolic diseases such as atrial fibrillation, myocardial infarction and venous thromboembolism. It is particularly important for the neurosurgeon to be familiar with this topic in order to balance risks between bleeding and thrombosis in association with surgery. This review provides an overview of the ‘cell-based’ theory of coagulation and the technique of rotational thromboelastograpy to measure whole-blood coagulation. We discuss current management strategies related to haemorrhage control, haemostasis in polytrauma, and the elective and emergency peri-operative management of aspirin, thienopyridines, warfarin and the novel oral anticoagulants (rivaroxaban, dabigatran, apixaban). Finally, the current evidence regarding neurosurgical thromboprophylaxis is reviewed.
Introduction
Coagulation will always be pivotal to the neurosurgeon. The finite space of the cranium mandates an acute concern to minimise the risk of haemorrhage, no matter how small. Much work has been undertaken recently that has improved our understanding of coagulation and this review article covers some of these critical advances. The modern ‘cell-based’ theory of haemostasis is discussed, along with new near-patient techniques to assess whole-blood coagulation. Beyond theory, we review changes to the acute management of haemostasis in the polytrauma patient, and the neurosurgical management of common antiplatelet agents (aspirin, clopidogrel, prasugrel and ticagrelol) warfarin and the novel oral anticoagulants (NOACs). Finally, the current evidence around thromboprophylaxis is reviewed.
Modern theory of coagulation
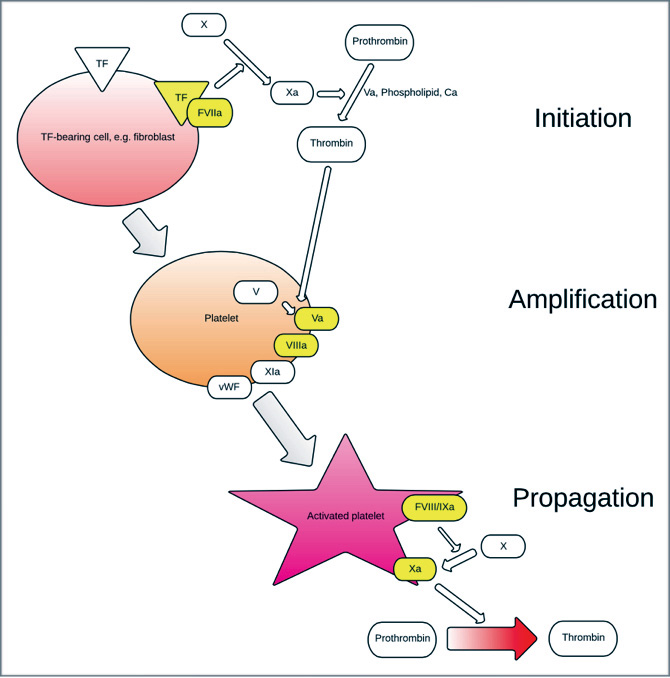
The classical model of clotting has been a ‘cascade’ of clotting factors associated with platelets in which there is a tissue-factor (TF) -initiated ‘extrinsic pathway’ and a contact-activated ‘intrinsic pathway.’ Undoubtedly, this has proven an accurate model to explain laboratory clotting assays such as prothrombin time (PT) and activated partial thromboplastin time (aPTT), however, a more modern theory has evolved that includes cellular and molecular components that better explains in-vivo haemostasis. Coagulation is theorised to be a process regulated by the properties of particular cell surfaces and occurs in three overlapping processes: initiation, amplification and propagation22 (Figure 1).
A breach in the wall of a blood vessel allows plasma to contact TF-bearing extra-vascular cells such as the fibroblast. TF is an integral membrane protein and structurally unrelated to other coagulation proteins.27 It remains localised to membrane of the cell in which it was synthesised and is neither expressed nor contained on normal circulating unactivated platelets. If this procoagulant stimulus is of sufficient strength, initiation commences on the surface of these TF-bearing cells. Factor VII is key, combining with TF to form a FVIIa/TF complex that activates factors X (itself activating factor V) and factor XI. Activated factor X (Xa) in combination with factor V, phospholipid and calcium (so-called prothrombinase) produce small volumes of thrombin (factor IIa) from the activation of Prothrombin (factor II).
In the amplification phase, platelets that previously adhered to extravascular collagen (by polymerised von Willebrand factor (vWF) released from endothelium), become partially activated. Factors Xa, IXa and thrombin induce further activation by adhering to platelet surface glycoproteins and other receptors. Platelets accumulate activated cofactors (Va, VIIIa, XIa and vWF) on their surface,29 amplifying the process. Activated platelets, with surface-bound Va and VIIIa, provide the surface for production of thrombin on a larger scale.
In the propagation phase, factor IXa diffuses from TF-bearing cells to the activated platelet surface, enabling the formation of active protease tenase (FVIIIa/IXa). Thrombin is the one of the primary activating factors from the small amount released during the initiation phase, causing back-activation of several clotting factors and platelets. Tenase activates factor X on the platelet surface, forming a complex with its co-factor to produce more prothrombinase (FXa/FVa). The activated platelet-surface FXa/Va promotes the development of haemostatic volumes of thrombin (so called thrombin ‘burst’) and the subsequent fibrin polymerisation necessary for coagulation. As well as activation of several clotting factors and platelets, thrombin is also involved in regulation of the haemostatic pathways by its activation of thrombomodulin and plasminogen.
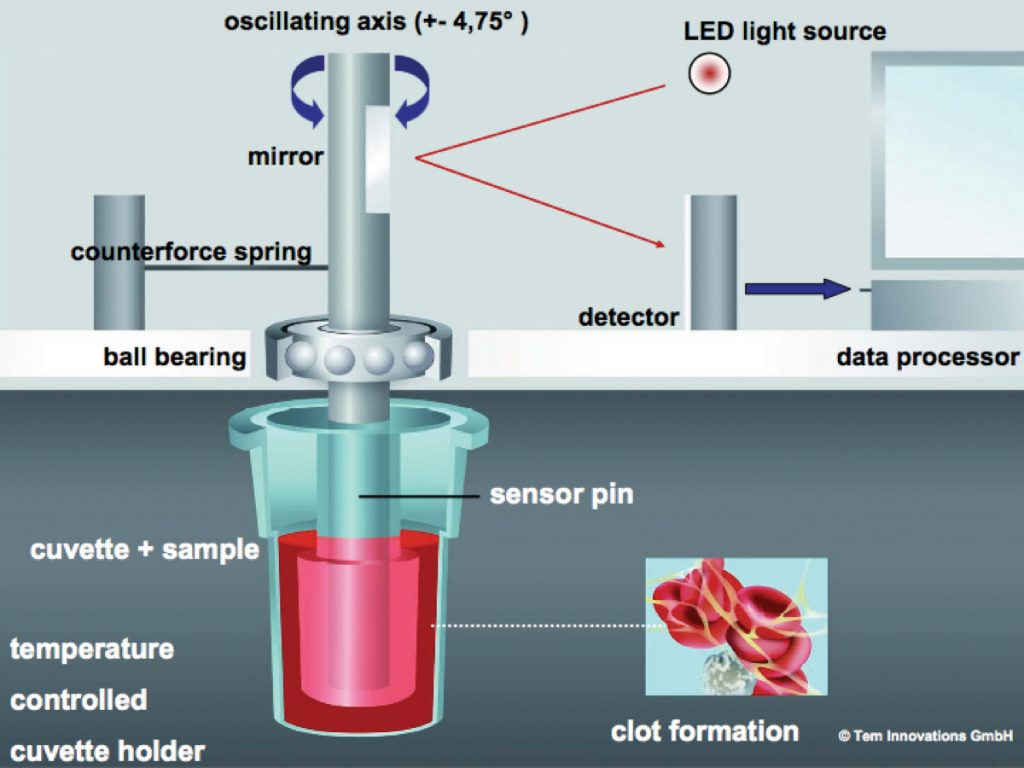
New technology for coagulation assessment: Rotational Thromboelastometry
As our understanding of coagulation has evolved, so have newer methods of monitoring dysfunctional coagulation. Standard laboratory tests of coagulation are performed on citrated plasma samples and include aPTT and PT. These tests are not affected either by platelet function or the surface properties of the whole haemostatic constitution as discussed previously. Since the advent of the cell-based model of coagulation there has been some concern over the appropriateness of the standard laboratory tests in the assessment of clotting due to the absence of the effect of any cell surface or platelet effects. Moreover, coagulation assays are performed using an excess of calcium and TF to stimulate clotting and thus are designed only to detect hypocoagulation that is due to factor or fibrin defects or deficiency. In many clinical situations it is not possible to determine the cause of abnormal haemostasis using these routine tests. These limitations have led to a growing interest into assays of coagulation that test haemostasis in whole blood, and the development of near-patient testing techniques.
Thromboelastography (TE) was first described in 1948 by Hartert21 as a means to monitor clot development by measuring its visco-elastic properties. It is now well established in the assessment of whole blood coagulation and in routine use across a range of surgical and anaesthetic specialties.5,25,34,36,37 TE has been refined by two companies into TEG® (Haemoscope Corporation, Niles, IL, USA) and rotational thromboelastography (ROTEM®, by © Tem International GmbH, Munich, Germany). Using the latter as an example, the technology employs a rotating shaft, connected to a spring that allows a computer to calculate changes in elasticity by detecting shifts in reflected light via an optical sensor. A disposable pin is connected to the tip of the shaft, this is inserted into a blood filled disposable cuvette. The exact position of the rotating shaft is used to calculate the change in elasticity of the blood being tested. Figure 2 illustrates this arrangement and a sample trace is seen in Figure 3. The definitions of the parameters reported in ROTEM® analysis are also given in Figure 3. It is beyond the scope of this article to provide detailed guidelines for the interpretation of such traces, but in essence the analysis is carried out along the time axis (left to right). Comparison between the measurements obtained and normal values can aid the clinician in diagnosing the underlying cause for haemorrhage. For example, abnormal clot formation prolongs the clot formation time (CFT) and often reduces the maximum clot firmness (MCF). If the CFT is affected more than MCF this implies a polymerisation disorder whereas if MCF is reduced with a normal CFT there is likely to be a deficiency in a clottable substrate (fibrinogen and/or platelets). Pathologic fibrinolysis is detected by shortening of the clotting time (CT) or a rise in the maximum lysis (ML). Different ROTEM® assays can also detect heparin effects.
As an ex-vivo test, ROTEM® provides information on the rate and quality of the interaction between the solid components of blood that result in fibrin polymerisation and thus the kinetics of clot formation and strength, and also subsequent fibrinolysis. As such, ROTEM® is considered to be a more sensitive test of haemostasis than standard laboratory clotting tests.
One of the greatest clinical benefits of this technology is in the definition of the coagulopathy to factor, platelet or fibrinogen deficiencies. The benefit of this rapid near-patient test has been clearly documented in a variety of fields including major trauma,19 neurosurgery17,25 and intensive care.2 It is likely that, as greater awareness of the cell-based theory of coagulation occurs, there will be greater surgical interest in TE as a better means of assessing whole blood coagulation and the optimum means by which correction can be rapidly achieved.
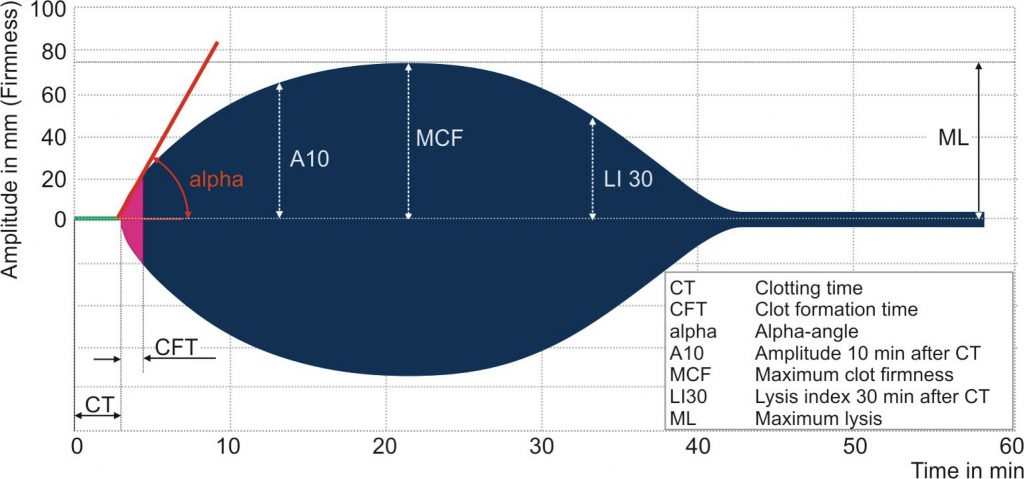
Coagulopathy in major trauma and haemorrhagic shock
Thromboelastography technologies have been integrated into the management of the patient with exsanguinating trauma. The treatment of such patients has rapidly evolved over the last twenty years. Prior strategies had incorporated aggressive and definitive surgical control of bleeding, aggressive crystalloid fluid resuscitation, without particular focus on either coagulopathy or management of the inflammatory response. Much has changed.18 Current strategies employ early ‘damage control’ surgery (including topical haemostatic agents and endovascular techniques) with definitive surgery after completion of resuscitation, early empiric (or TE guided) administration of clotting factors to address coagulopathy, blood component resuscitation with permissive hypotension until control of haemorrhage and minimal use of fluids that exacerbate inflammation during resuscitation. These changes have been driven by research into areas including microcirculatory and inflammatory changes in shock that are outside the remit of this article, but progress in the understanding of coagulation has also been critical.
Coagulopathy in severe trauma is common, occurring in as many as one in four patients,6 and is initiated by the maladaptive response to the combination of severe shock and tissue trauma. This combination initiates multiple poorly-understood pathological mechanisms resulting in systemic anticoagulation and fibrinolysis.18 Previous theories have included hypothermia, acidaemia and consumption of coagulation factors as the underlying causes, although it is now clear that these, although important, are not central to the pathologic mechanisms, particularly in the acute phase. It is more likely that the dilution of clotting factors either consequent to auto-resuscitation or iatrogenic (with packed red cell or crystalloid resuscitation) is contributory,35 compounded by both the inflammatory response18 and platelet dysfunction.46
Better understanding of the detrimental effects of red cell and crystalloid resuscitation have driven the development of institutional ‘massive transfusion protocols,’ that include plasma, platelet and cryoprecipitate components. Difficulties in the provision of thawed fresh frozen plasma in the acute setting can be circumvented by the use of freeze-dried plasma,28 allowing for rapid and high volume plasma transfusion, although this is not currently readily available. However, whilst massive transfusion protocols are likely only to alleviate further iatrogenic exacerbation of traumatic coagulopathy, other products are intended to actively reverse the coagulopathy. Recent areas of interest have included fibrinogen concentrate (readily available in other parts of Europe), recombinant factor VIIa prothrombin complex concentrate (PCC) and tranexamic acid. The last of these has demonstrated a significant reduction in mortality in general trauma patients in the CRASH-2 trial9 and work is ongoing on the CRASH-3 trial into isolated traumatic brain injury.11
Antiplatelet and anticoagulation agents:
1) Antiplatelet agents:
Oral anticoagulant and/or antiplatelet agents are commonly prescribed for primary or secondary prevention of stroke, myocardial infarction, peripheral vascular disease and VTE. However, with their significant reduction in thromboembolic events, comes an increased risk of intracerebral haemorrhage (7-10 fold for oral anticoagulation), which is often larger at initial presentation and has a greater risk of extension.14
Aspirin is a very commonly prescribed antiplatelet, which irreversibly binds to cyclooxygenase-1 and inhibits the production of thromboxane A2 (TXA2), adversely affecting platelet function throughout its life (7-10 days). It is metabolised rapidly to an ineffective metabolite, and thus all new platelets produced thereafter have normal function. Therefore, discontinuing aspirin 10 days prior to elective neurosurgery is sufficient to ensure normal platelet function. Emergency correction is achieved by a single unit of platelets, the adult therapeutic dose (ATD).
The thienopyridine drugs clopidogrel and prasugrel are newer antiplatelet agents with greater efficacy than aspirin, which probably leads to a higher degree of morbidity and mortality in the neurosurgical patient.7 They are prodrugs and undergo hepatic metabolism (by cytochrome P450) to their functional metabolite. They irreversibly bind to P2Y12 subtype of the platelet ADP receptor, inhibiting the GPIIb/IIIa complexes, fibrinogen binding and P-selectin expression.23 Another different P2Y12 inhibitor (ticagrelor) is also being increasingly used in modern arterial dysfunction management. In the elective setting, normal platelet function can be expected 10 days after discontinuation.45 As platelet dysfunction is more severe than that of aspirin, in neurosurgical emergencies the prescription of 2 units of platelets has been suggested.4 Other groups have suggested platelet transfusions do not achieve correction in all patients on clopidgrel40. Although there is an absence of high quality evidence it is reasonable to undertake emergency surgery with caution subsequent to a 2 unit platelet transfusion.
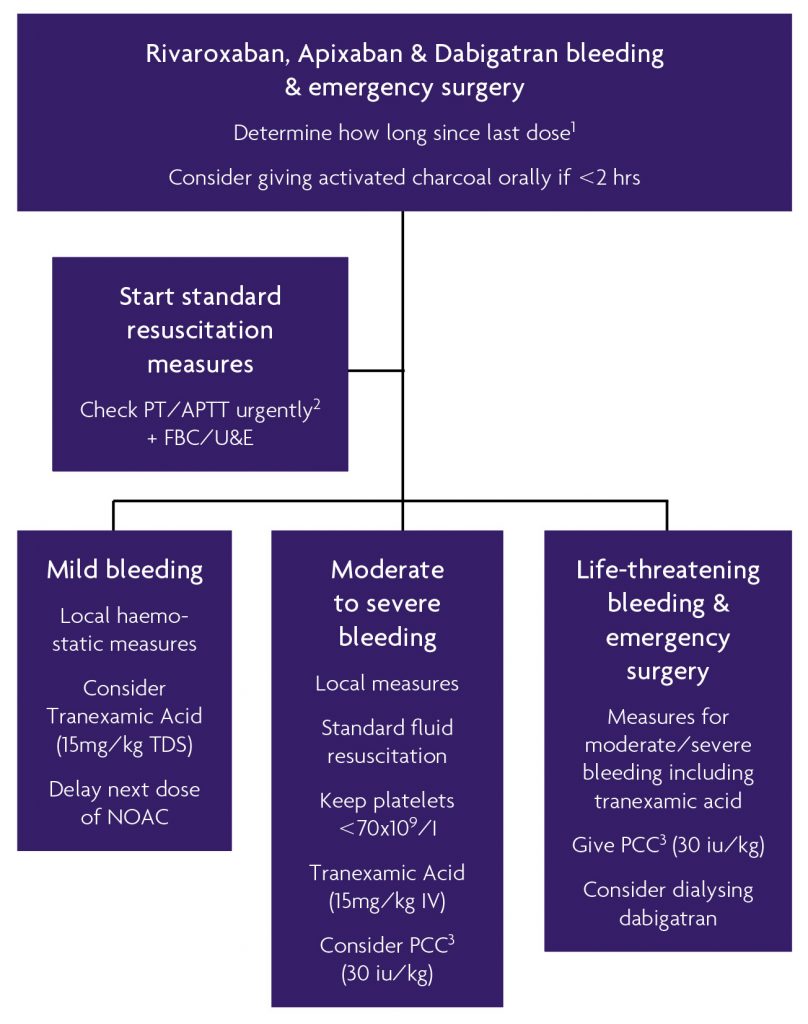
2) Classical anticoagulants:
Warfarin, a commonly prescribed anticoagulant, inhibits vitamin K epoxide reductase and thus antagonises vitamin K dependent factors (FII, FVII, FIX, FX, protein C and S). The reversal strategies are twofold: improved regeneration by vitamin K supplementation and direct replacement of the depleted coagulation factors. The former is slow, with intravenous administration only beginning to reduce the INR from two hours, and has an unpredictable response. It is appropriate for ‘elective’ correction as a normal INR can be achieved in most cases within 24 hours. Intravenous doses of 5-10mg of vitamin K are normally advocated.3 Having said that, hospital protocols will often suggest merely stopping the warfarin approximately five days before the planned procedure, with monitoring of the INR. Consideration should be made in such cases as to the need for bridging therapy. This usually takes the form of either low molecular weight heparin or unfractionated heparin, and the decision to prescribe bridging therapy is dictated by stratifying the thromboembolic risk to the patient.
In emergency situations, it is necessary to replace the depleted coagulation factors more rapidly. Fresh frozen plasma (FFP) can be effective but has several limitations: very variable levels of vitamin K dependent factors, thawing takes time causing inevitable delays and large volumes required can cause overload and congestive cardiac failure, particularly in elderly patients. FFP is now actively discouraged for emergency reversal of vitamin K antagonist drugs such as warfarin. Consequently, four factor prothrombin complex concentrate (PCC) is now recommended by haematology guidelines (BCSH). These have predictable, higher levels of vitamin K dependent factors and thus allow almost immediate correction of associated coagulopathy and smaller transfusion volume. The elimination half-life of some factors in PCC is short, and therefore it is recommended that vitamin K (5-10mg IV) is concurrently prescribed to avoid the rebound to an anticoagulated state.4 Post PCC INR should be monitored to detect this potential rebound effect.
3) Direct New Oral Anticoagulants (NOACs)
The NOACs are either direct thrombin (dabigatran) or direct factor Xa (rivaroxaban and apixaban) inhibitors, and are used largely in the prevention of systemic embolisation in non-valvular atrial fibrillation or the treatment of venous thromboembolic (VTE) disease. They benefit from predicable pharmacokinetic and pharmacodynamic profiles compared with alternatives such as warfarin,13 do not require laboratory monitoring and are equivalent or have significantly improved efficacy to warfarin in preventing stroke or embolic disease.8,32 Significantly however, there are no current specific reversal agents and thus these agents are problematic for neurosurgeons!15
Apixaban and rivaroxaban have a half-life of 7-14 hours33,42 and are predominantly liver excreted (65-75%). Dabigatran has a half-life of 12-17 hours39 and is 80% excreted in the urine.
Whilst there is no requirement to monitor these agents in routine practice, in the emergency situation, assessment of the aPTT and PT can be useful only to check if any active drug is not present, when in the normal range. The aPTT is increased by dabigatran, rivaroxaban and apixaban, however the relationships are not straightforward. The PT and INR are poorly sensitive to dabigatran and not suitable for monitoring.39 Conversely, PT correlates better with rivaroxaban and apixaban plasma concentration, but is dependent on PT reagents used and is not very linear.
If elective neurosurgery is required whilst the patient is taking NOACs, then one should discontinue the medications prior to surgery. Fawole et al.13 have suggested the following guideline for termination of the NOAC prior to elective surgery (Table 1) based on a number of studies.
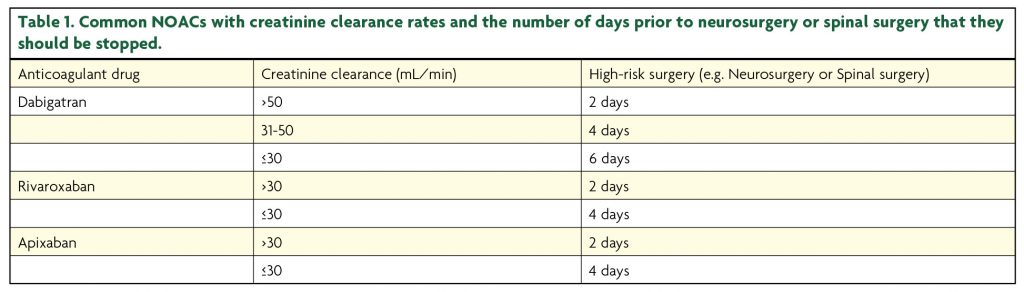
The optimal time to re-start NOACs after neurosurgery is not defined. Consensus guidelines suggest it can be re-started once the surgical bleeding risk is under control in the early post-operative period.41 It has been suggested that re-commencement at 24-48 hours post-operatively is reasonable and some have suggested that clinicians might consider a re-introduction at half the patients’ normal dose for the first day.38 However, where some thromboprophylaxis is required, the use of low dose low molecular weight heparin is probably sensible and licenced.
If patients suffer significant intra-cerebral haemorrhage whilst prescribed a NOAC, they should be considered for intensive care treatment and an accurate drug history must be obtained including the time of last NOAC dose. If within two hours, charcoal administration may be helpful. There are no specific reversal agents beyond studies in rat models,24 although clinicians might consider supportive therapies such as fluid resuscitation or packed red-cell transfusions.43 In addition, recombinant factor VII (rVIIa, novoseven) and three or four factor PCCs and Factor VIII inhibitor bypassing activity (FEIBA or activated four-factor prothrombinase complex concentrate) have also been suggested, however the evidence is not strong.10,12,26 Haemodialysis can be considered to accelerate the removal of dabigatran in life-threatening cases. Rivaroxaban and apixaban are more highly plasma protein bound and haemodialysis is not useful in these cases. Our current protocol for the reversal of NOACs is demonstrated in figure 4. We do not advocate the use of rVIIa as there has been a number of adverse arterial events in previous off licence trials. We do suggest using tranexamic acid for moderate to life-threatening bleeds, but not FFP as the factor concentrations are so unpredictable.
Peri-operative venous thromboprophylaxis (TP)
All surgical patients mandate a decision regarding the timing of peri-operative deep venous thromboprophylaxis. Neurosurgical patients are particularly complex in that even a small, induced haemorrhage secondary to thromboprophylaxis can be fatal or cause severe morbidity. However, given the long surgical times and often poor mobility post-operatively, the patients are at significant risk of VTE. Decision-making about when to commence TP is challenging. Options available to the surgeon broadly include unfractionated heparin (UFH) or low molecular weight heparin (LMWH) for chemical prophylaxis, and intermittent pneumatic compression, venous foot-pumps and anti-embolism stockings as mechanical options.
NICE guidance (2010) on TP has suggested neurosurgical patients who are at increased risk of VTE should commence mechanical prophylaxis at admission and this should continue for the duration of any impaired mobility.30 Pharmacological prophylaxis (UFH or LMWH) should be added for any patient without risk of major bleeding, but this should be considered at an individual patient level. There is specific advice that pharmacological TP is not prescribed to patients with unsecured, ruptured cranial or spinal vascular malformations or haemorrhage in the unstable patient.
UFH has a short half-life (45-90 mins) and thus requires continuous intravenous infusion to achieve therapeutic anticoagulation, although it can also be prescribed as a twice or thrice daily subcutaneous injection for prophylaxis. With intravenous prescriptions of UFH it is necessary to monitor the therapeutic activity with regular aPTT measurement as the response can be highly variable. Owing to its short half-life, correction can be rapidly achieved by the termination of the infusion. It is therefore useful for short-term therapeutic anticoagulation in the patient with a high risk of both thromboembolism and haemorrhagic complications. In situations requiring emergency correction, protamine sulphate is very effective.4 LMWH has a longer half-life (6-12 hours) and is therefore more convenient for the patient. It is not necessary to monitor its therapeutic activity in routine clinical situations, although this is possible with anti-Xa assays, and should be considered in those with renal impairment (GFR <30ml/min), the morbidly obese patient and pregnancy. It is, however, not totally reversible. Protamine can reverse 40-60% of the anti-FXa activity, at the recommended dose of 1mg per 1mg of LMWH administered in the previous four hours.1,44
Significant recent work into TP in neurosurgical patients has been undertaken by Hamilton et al.,20 publishing a systematic review and meta-analysis of eight randomised controlled trials. Six studies compared either UFH or LMWH (low molecular weight heparin) with or without mechanical prophylaxis, with placebo or mechanical prophylaxis. Two studies compared UFH plus mechanical prophylaxis with LWMH plus mechanical prophylaxis. They found that heparin prophylaxis reduced the risk of symptomatic and asymptomatic VTE by 42%, but with an insignificant increase in the rate of intracerebral haemorrhage (ICH), and a statistically significant doubling of minor haemorrhages (e.g. extracranial haemorrhage). These corresponded to a number needed to treat (NNT) to prevent a VTE of 11, and a number needed to harm (NNH) of 143 for ICH and 36 for other minor bleeding. Clinicians should be cogent to the impact of the events however, most would consider a screened finding of a distal lower limb deep venous thrombosis (DVT) less of a burden to the patient than a proximal or symptomatic DVT, or a pulmonary embolus. The ratio of screen-detected events to symptomatic events is in the region of 10-20%.16 This is important as only two RCTs in the aforementioned meta-analysis distinguished such clinically significant VTEs from screen-detected DVTs. However, with all such trials of anticoagulants, which report asymptomatic DVT, it cannot be predicted who will go on to develop significant VTE events. Ultimately, Hamilton et al. state that for every 1000 elective cranial neurosurgical patients, 56 clinically asymptomatic distal DVTs will be prevented, 35 proximal DVTs/PEs would be prevented (of which 9-18 would be symptomatic). Conversely, seven patients could suffer an ICH. Therefore, the decision in these cases ultimately rests on the risk of a statistically significant reduction in the risk of symptomatic VTE versus the clinical significance of an intracerebral haemorrhage.
Conclusions
The modern neurosurgeon has witnessed the emergence of new challenges in coagulation. These include: polytrauma patients, the significant increase of elderly neurosurgical patients who take antiplatelet agents, increased numbers of patients anticoagulated with warfarin, and the increasing numbers of patients taking NOACs. In addition whilst NOACs have reliable pharmacokinetics, they lack an accurate method to measure their effects and clear options for timely reversal of their effects. However, the stroke prevention trials of NOACs have shown significant reductions in intracranial bleeding over warfarin; trials of reversal agents are ongoing. Fortunately, advances in both our understanding of the theory behind coagulation, and better methods for its assessment with rapid near-patient testing provide us with an armamentarium with which we can address some of these challenges.
References
- Adler BK. Unfractionated heparin and other antithrombin mediated anticoagulants. Clin Lab Sci 2004;17:13- 117.
- Anderson L, Quasim I, Soutar R, Steven M, Macfie A, Korte W. An audit of red cell and blood product use after the institution of thromboelastometry in a cardiac intensive care unit. Transfus Med 2006;16:31-39. https://doi.org/10.1111/j.1365-3148.2006.00645.x
- Ansell J, Hirsh J, Hylek E, Jacobson A, Crowther M, Palareti G, et al. Pharmacology and management of the vitamin K antagonists: American College of Chest Physicians Evidence-Based Clinical Practice Guidelines (8th Edition). Chest 2008;133:160S-198S. https://doi.org/10.1378/chest.08-0670
- Beshay JE, Morgan H, Madden C, Yu W, Sarode R. Emergency reversal of anticoagulation and antiplatelet therapies in neurosurgical patients. Journal of Neurosurgery 2010;112:307-318. https://doi.org/10.3171/2009.7.JNS0982
- Bischof D, Dalbert S, Zollinger A. Thrombelastography in the surgical patient. Minerva Anestesiologica 2010;76:131-137.
- Brohi K, Singh J, Heron M, Coats T. Acute traumatic coagulopathy. Journal of Trauma 2003;54:1127-1130. https://doi.org/10.1097/01.TA.0000069184.82147.06
- Campbell PG, Yadla S, Sen AN, Jallo J, Jabbour P. Emergency Reversal of Clopidogrel in the Setting of Spontaneous Intracerebral Hemorrhage. World Neurosurgery 2011;76:100-104. https://doi.org/10.1016/j.wneu.2011.02.010
- Connolly SJ, Ezekowitz MD, Yusuf S, Eikelboom J, Oldgren J, Parekh A, et al. Dabigatran versus Warfarin in Patients with Atrial Fibrillation. N Engl J Med 2009;361:1139-1151. https://doi.org/10.1056/NEJMoa0905561
- CRASH-2 trial collaborators, Shakur H, Roberts I, Bautista R, Caballero J, Coats T, et al. Effects of tranexamic acid on death, vascular occlusive events, and blood transfusion in trauma patients with significant haemorrhage (CRASH-2): a randomised, placebo-controlled trial. Lancet 2010;376:23-32. https://doi.org/10.1016/S0140-6736(10)60835-5
- Dager WE, Gosselin RC, Roberts AJ. Reversing dabigatran in life-threatening bleeding occurring during cardiac ablation with factor eight inhibitor bypassing activity. Crit Care Med 2013;41:e42-6. https://doi.org/10.1097/CCM.0b013e31827caaa3
- Dewan Y, Komolafe EO, Mejía-Mantilla JH. Perel P. CRASH-3-tranexamic acid for the treatment of significant traumatic brain injury: study protocol for an international randomized, double-blind, placebo-controlled trial. Trials:2012. https://doi.org/10.1186/1745-6215-13-87
- Eerenberg ESE, Kamphuisen PWP, Sijpkens MKM, Meijers JCJ, Buller HRH, Levi MM. Reversal of rivaroxaban and dabigatran by prothrombin complex concentrate: a randomized, placebo-controlled, crossover study in healthy subjects. Circulation 2001;124:1573-1579. https://doi.org/10.1161/CIRCULATIONAHA.111.029017
- Fawole A, Daw HA, Crowther M. Practical management of bleeding due to the anticoagulants dabigatran, rivaroxaban, and apixaban. Cleveland Clinic Journal of Medicine 2013;80:443-51. https://doi.org/10.3949/ccjm.80a.13025
- Franke CL, De Jonge J, Van Swieten JC, De Coul A. Intracerebral hematomas during anticoagulant treatment. Stroke 1991;21:726-30. https://doi.org/10.1161/01.STR.21.5.726
- Garber ST, Sivakumar W, Schmidt RH. Neurosurgical complications of direct thrombin inhibitors-catastrophic hemorrhage after mild traumatic brain injury in a patient receiving dabigatran. Journal of Neurosurgery 2012;116:1093-1096. https://doi.org/10.3171/2012.2.JNS112132
- Geerts WH, Pineo GF, Heit JA, Bergqvist D, Lassen MR, Colwell CW, et al. Prevention of venous thromboembolism: the Seventh ACCP Conference on Antithrombotic and Thrombolytic Therapy. Chest 2004;126:338S-400S. https://doi.org/10.1378/chest.126.3_suppl.338S
- Görlinger K: Goal-directed therapy in trauma induced coagulopathy and focus on traumatic brain injury. Reviews in Health Care 2014;4:17-29. https://doi.org/10.7175/rhc.v4i3S.877
- Gruen RL, Brohi K, Schreiber M, Balogh ZJ, Pitt V, Narayan M, et al.: Haemorrhage control in severely injured patients. The Lancet 2012;380:1099-1108. https://doi.org/10.1016/S0140-6736(12)61224-0
- Schochl H, Nienaber U, Hofer G, Voelckel W, Jambor C Scharbert G, et al. Goal-directed coagulation management of major trauma patients using thromboelastometry (ROTEM)-guided administration of fibrinogen concentrate and prothrombin complex concentrate. Crit Care 2012;14:R55-R55. https://doi.org/10.1186/cc8948
- Hamilton MG, Yee WH, Hull RD, Ghali WA. Venous thromboembolism prophylaxis in patients undergoing cranial neurosurgery: a systematic review and meta-analysis. Neurosurgery 2011;68:571-581. https://doi.org/10.1227/NEU.0b013e3182093145
- Hartert H: Blutgerinnungsstudien mit der Thrombelastographie, einem neuen Untersuchungsvefahren. Klin Wochenschr 1948;26:577-583. https://doi.org/10.1007/BF01697545
- Hoffman M, Monroe DM. A cell-based model of hemostasis. Thrombosis and Haemostasis 2001;85:958-965. https://doi.org/10.1055/s-0037-1615947
- Kam PCA, Nethery CM. The thienopyridine derivatives (platelet adenosine diphosphate receptor antagonists), pharmacology and clinical developments. Anaesthesia 2003;58:28-35. https://doi.org/10.1046/j.1365-2044.2003.02960.x
- Lu G, DeGuzman FR, Hollenbach SJ, Karbarz MJ, Abe K, Lee G, et al. A specific antidote for reversal of anticoagulation by direct and indirect inhibitors of coagulation factor Xa. Nat Med 2013;19:446-451. https://doi.org/10.1038/nm.3102
- Luostarinen T, Silvasti-Lundell M, Medeiros T, Romani R, Hernesniemi J, Niemi T. Thromboelastometry during intraoperative transfusion of fresh frozen plasma in pediatric neurosurgery. J Anesth 2012;26:770-774. https://doi.org/10.1007/s00540-012-1400-z
- Marlu R, Hodaj E, Paris A, Albaladejo P, Crackowski JL, Pernod G. Effect of non-specific reversal agents on anticoagulant activity of dabigatran and rivaroxaban: a randomised crossover ex vivo study in healthy volunteers. Thrombosis and Haemostasis 2012;108:217-224. https://doi.org/10.1160/TH12-03-0179
- Martin DM, Boys CW, Ruf W. Tissue factor: molecular recognition and cofactor function. FASEB J 1995;9:852-859. https://doi.org/10.1096/fasebj.9.10.7615155
- Martinaud C, Ausset S, Deshayes AV, Cauet A, Demazeau N, Sailliol A. Use of freeze-dried plasma in French intensive care unit in Afghanistan. J Trauma 2011;71:1761-4- discussion 1764-5. https://doi.org/10.1097/TA.0b013e31822f1285
- Monroe DM, Hoffman M, Roberts HR: Transmission of a procoagulant signal from tissue factor-bearing cell to platelets. Blood Coagul Fibrinolysis 7:459-464, 1996 https://doi.org/10.1097/00001721-199606000-00005
- NICE: Venous thromboembolism: reducing the risk. National Institute for Health and Care Excellence 2010;CG92.
- Pabinger I, Brenner B, Kalina U, Knaub S, Nagy A, Ostermann H, et al. Prothrombin complex concentrate (Beriplex P/N) for emergency anticoagulation reversal: a prospective multinational clinical trial. J Thromb Haemost 2008;6:622-631. https://doi.org/10.1111/j.1538-7836.2008.02904.x
- Patel MR, Mahaffey KW, Garg J, Pan G, Singer DE, Hacke W, et al. Rivaroxaban versus warfarin in nonvalvular atrial fibrillation. N Engl J Med 2011;365:883-891. https://doi.org/10.1056/NEJMoa1009638
- Raghavan N, Frost CE, Yu Z, He K, Zhang H, Humphreys WG, et al. Apixaban metabolism and pharmacokinetics after oral administration to humans. Drug Metabolism and Disposition 2008;37:74-81. https://doi.org/10.1124/dmd.108.023143
- Reinhöfer M, Brauer M, Franke U, Barz D, Marx G, Lösche The value of rotation thromboelastometry to monitor disturbed perioperative haemostasis and bleeding risk in patients with cardiopulmonary bypass. Blood Coagul Fibrinolysis 2008;19:212-219. https://doi.org/10.1097/MBC.0b013e3282f3f9d4
- Rourke C, Curry N, Khan S, Taylor R, Raza I, Davenport R, et al. Fibrinogen levels during trauma hemorrhage, response to replacement therapy, and association with patient outcomes. J Thromb Haemost 2012;10:1342-1351. https://doi.org/10.1111/j.1538-7836.2012.04752.x
- Schöchl H, Nienaber U, Maegele M, Hochleitner G, Primavesi F, Steitz B, et al. Transfusion in trauma: thromboelastometry-guided coagulation factor concentrate-based therapy versus standard fresh frozen plasma-based therapy. Crit Care 2011;15:R83-R83. https://doi.org/10.1186/cc10078
- Schöchl H, Solomon C, Traintinger S, Nienaber U, Tacacs-Tolnai A, Windhofer C, et al. Thromboelastometric (ROTEM) findings in patients suffering from isolated severe traumatic brain injury. Journal of Neurotrauma 2011;28:2033-2041. https://doi.org/10.1089/neu.2010.1744
- Schulman SS, Crowther MAM: How I treat with anticoagulants in 2012: new and old anticoagulants, and when and how to switch. Blood 2012;119:3016-3023. https://doi.org/10.1182/blood-2011-10-378950
- Stangier J, Rathgen K, Stähle The pharmacokinetics, pharmacodynamics and tolerability of dabigatran etexilate, a new oral direct thrombin inhibitor, in healthy male subjects. British journal of of Clinical Pharmacology 2007;64:292-303. https://doi.org/10.1111/j.1365-2125.2007.02899.x
- Taylor G, Osinski D, Thevenin A, Devys J-M. Is platelet transfusion efficient to restore platelet reactivity in patients who are responders to aspirin and/or clopidogrel before emergency surgery? Journal of Trauma and Acute Care Surgery 2013;74:1367-1369. https://doi.org/10.1097/01586154-201305000-00027
- Turpie A, Kreutz R, Llau J, Norrving B. Management consensus guidance for the use of rivaroxaban-an oral, direct factor Xa inhibitor. Thrombosis and Haemostasis 2012;108:877-887. https://doi.org/10.1160/TH12-03-0209
- Mueck W, Becka M, Kubitza D, Voith B, Zuehlsdorf M. Population model of the pharmacokinetics and pharmacodynamics of rivaroxaban–an oral, direct factor xa inhibitor–in healthy subjects. Int J Clin Pharmacol Ther 2007;45:335-344. https://doi.org/10.5414/CPP45335
- Wann LS, Curtis AB, Ellenbogen KA, Estes NAM, Ezekowitz MD, Jackman WM, et al. 2011 ACCF/AHA/HRS focused update on the management of patients with atrial fibrillation (update on dabigatran): a report of the American College of Cardiology Foundation/American Heart Association Task Force on practice guidelines. J Am Coll Cardiol 2011;57;1330-1337. https://doi.org/10.1161/CIR.0b013e31820f14c0
- Warkentin TE, Crowther M. Reversing anticoagulants both old and new. Can J Anaesth 2002;49:S11-S25.
- Weber AA, Braun M, Hohlfeld T, Schwippert B, Tschöpe D, Schrör Recovery of platelet function after discontinuation of clopidogrel treatment in healthy volunteers. Br J Clin Pharmacol 2001;52:333-336. https://doi.org/10.1046/j.0306-5251.2001.01453.x
- Wohlauer MV, Moore EE, Thomas S, Sauaia A, Evans E, Harr J, et al. Early Platelet Dysfunction: An Unrecognized Role in the Acute Coagulopathy of Trauma. Journal of the American College of Surgeons 2012;214:739-746. https://doi.org/10.1016/j.jamcollsurg.2012.01.050