Throughout life, the adult mammalian brain continuously generates new neurons that become integrated into pre-existing neuronal networks. Under physiological conditions adult neurogenesis is restricted to two neurogenic niches, i.e. the subventricular zone (SVZ), which generate interneurons that will integrate into the olfactory bulb, and the hippocampal dentate gyrus (DG), where new granule cells of the DG (DGCs) are continuously produced.
Newborn DGCs derive from neural progenitor cells (NPCs) of the DG subgranular zone through a process that lasts several weeks to reach maturity (Figure 1).
During the first week of development, early neuroblasts undergo radial migration toward the inner granule cell layer. Axon and dendrite formation starts during the second week of development and coincides with afferent synaptogenesis with GABAergic neurons. Glutamatergic functional afferents begin to establish during the third week of maturation and increase their strength in the following week. Structural and functional maturation of new DGCs is completed after 6-8 weeks.
During this maturation process, DG newborn neurons display enhanced synaptic plasticity properties (i.e.increased intrinsic excitability and reduced sensitivity to GABAergic inhibition) compared with that of preexisting mature DGCs. Remarkably, newborn neurons feature a specialised form of long term potentiation (LTP) that is distinguishable from that of mature DGCs for its reduced sensitivity to GABAergic inhibition1. Such peculiar electrophysiological properties apparently confer to newborn neurons a key role in regulating hippocampal function and cognition.
The DG plays a pivotal role in cognition. Experimental and computational studies indicate that newborn neurons in the DG are essential for hippocampal function. In fact, there is increasing evidence that maturing newborn neurons distinctively contribute to information processing in the DG and participate in the expression of specific forms of hippocampal-dependent learning and memory in rodents, including contextual learning, cognitive flexibility and pattern separation.
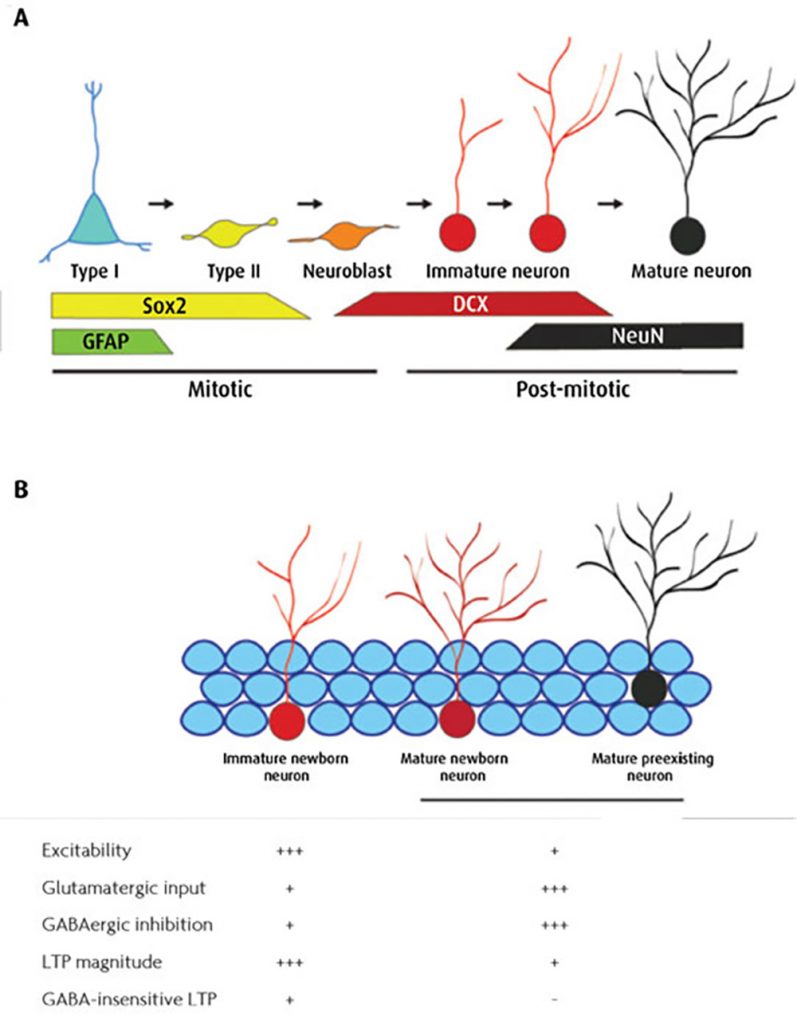
The impairment of adult neurogenesis has been observed in a variety of models relevant to neuropsychiatric diseases, such as major depression,schizophrenia and Alzheimer’s disease, and neurodevelopmental disorders, including Fragile X and Down syndrome (DS) (for reviews see 2,3). Furthermore, recent data in epileptic patients undergoing en bloc hippocampus resection indicate that low proliferation and differentiation capacities of adult hippocampal stem cells correlate with memory dysfunction4. However, to date, the evidence supporting a role for adult neurogenesis in specific diseases is still sparse and inconclusive.Moreover,it is still unclear whether impairment of adult neurogenesis associated with neurodegenerative and neurodevelopmental diseases is a primary event or a neuroadaptive response to complex pathological mechanisms. Nevertheless, independently of the origin of its impairment, one fundamental question is whether adult neurogenesis could be boosted and exploited for therapeutic purposes. Indeed, adult neurogenesis is highly sensitive to environmental stimuli, including environmental enrichment and exogenous molecules5. Thus, adult neurogenesis represents an attractive, though unexplored, opportunity to develop therapies for disease-associated cognitive impairment and might be particularly relevant for neurodevelopmental diseases with complex genetic etiologies, such as DS.
DS results from the trisomy of chromosome 21 in humans and is the leading cause of genetically defined intellectual disability. In the DS brain, suboptimal network architecture and altered synaptic communication through neurodevelopmental impairment represent key determinants of intellectual disabilities, which involve several cognitive domains, such as language, verbal and spatial learning and memory (reviewed in 2). Both hippocampal and prefrontal-related functions appear defective in DS subjects, although these defects apparently manifest at different ages. Hippocampal dysfunction occurs in DS children and adolescents, with impairment of spatial associative memory and sparing of the prefrontal-mediated reference memory6.
Over the past decades, several trisomic DS mouse models have been generated, recapitulating essential genetic and cognitive deficits of human DS (reviewed in 2,7). The results of studies conducted in such models have suggested that cognitive dysfunction is not due to gross neuroanatomical abnormalities, but rather derives from both changes in synaptic connectivity (e.g.excitation/ inhibition imbalance) and altered developmental neurogenesis during embryogenesis and the early postnatal period (reviewed in 2).
The widely used Ts65Dn mouse is a relevant model of DS,reproducing several phenotypic abnormalities of the human disease8. Studies using this model have reported that adult neurogenesis is reduced in both the adult DG and SVZ, and its stimulation using drugs, such as fluoxetine9 or lithium10,11, induces the proliferation of NPCs and the expression of discrete molecular markers of newborn neurons in both neurogenic niches. Specifically, it has been shown that treatment with fluoxetine for up to 24 days rescues the impaired hippocampal neurogenesis of young Ts65Dn mice,re-establishing levels of proliferating cells similar to euploid mice9.Beneficial effects on neurogenesis have also been obtained in the SVZ of 12 month-old Ts65Dn female mice fed with lithium-supplemented chow for 1 month10. Using the same model, we took one step further and we recently demonstrated that potentiation of dentate adult neurogenesis could translate into amelioration of hippocampal plasticity and behavioural performance in the multi-factorial context of DS11.We found that the number of DG newborn neurons is reduced and neurogenesis-dependent LTP is deficient in the DG of Ts65Dn mice. Notably, the lack of this specialised form of synaptic plasticity in trisomic mice correlates with the impaired performance of Ts65Dn in hippocampal-dependent behavioural tasks, such as the contextual fear conditioning and object location tasks11. To enhance neurogenesis, we treated 5-6 month old Ts65Dn mice with lithium, a mood-stabilising agent that specifically stimulates the proliferation of NPCs through the Wnt/Beta–catenin pathway. We found that DG adult neurogenesis could be restored to physiological levels after 4 weeks of lithium administration in the Ts65Dn trisomic mouse, resulting in the full recovery of DG synaptic plasticity and hippocampal-dependent cognitive functions.Lithium achieves these results by both enhancing NPC proliferation and favouring the integration of newborn neurons into the hippocampal circuit. Indeed, in trisomic mice, lithium stimulates NPC proliferation and increases the numbers of maturing DG newborn neurons without affecting fate determination and newborn neuron survival. Such neurons are functionally active, completely recover newborn neuron-dependent LTP, and are required to restore hippocampal-dependent learning and memory performance in lithium-treated trisomic mice.
Notably, we demonstrated that LTP was not rescued when lithium-treated trisomic and wild type mice were concomitantly administered the cytostatic drug temozolamide to inhibit NPCs proliferation,indicating that neurogenesis is required for LTP recovery. In this view line, short-term treatment (e.g. one week) with lithium fails to restore hippocampal synaptic plasticity in Ts65Dn mice.This rules out the involvement of other acute effects of lithium and further supports the notion that functional neurogenesis is specifically involved in reverting the hippocampal-dependent disease phenotype. Indeed, newborn neurons one-week old or younger do not show any spontaneous or evoked synaptic currents and are not integrated in the hippocampal circuits1.In fact,their enhanced synaptic plasticity properties start to be expressed 3-4 weeks after their birth and last until 6 weeks1.
Recovery of neurogenesis and newborn neuron-dependent LTP in Ts65Dn mice results in the specific rescue of behavioural performance in different hippocampal-dependent tasks, but not of working memory (i.e. T-maze). This is consistent with previous studies showing that only some forms of learning12,13, rely on hippocampal adult neurogenesis, while working memory is independent of adult neurogenesis14.Although we acknowledge that lithium may exert diverse pleiotropic effects, such as for example, modulation of brain myo-inositol levels, our data consistently support the view that amelioration of adult neurogenesis underlies lithium effects on behavioural performance in Ts65Dn mice. This indicates that restoring adult neurogenesis to physiological levels can resolve some crucial hippocampal-dependent cognitive defects typical of DS.In DS,hippocampal dysfunction is characterised by impaired spatial associative memory as detected by the CANTAB Paired-associates Learning6 and is particularly evident in very demanding tasks. Although these and other results (reviewed in 2,7) suggest a potential impairment of cognitive functions typically regulated by adult neurogenesis, such as pattern separation15 and cognitive flexibility16, further studies are required to evaluate in depth these specific domains in DS patients and related mouse models.
From the molecular point of view,we found that the Wnt/Beta-catenin pathway mediates the neurogenesis-promoting effects of lithium, as functional signalling through this pathway is required for lithium-induced proliferation of trisomic and wild type adult dentate NPCs in vitro. However, at present, we cannot rule out that other neurogenic pathways may underlie adult neurogenesis impairment in DS and may also be exploited from a therapeutic perspective. Further studies are warranted to identify abnormal neurogenic pathways in DS and/or their potential exploitation for therapies.
In summary, our findings11 strongly indicate that promoting adult neurogenesis may have profound impact on hippocampal synaptic plasticity and memory in DS. This is particularly relevant from the therapeutic perspective in view of the recent discovery that adult hippocampal neurogenesis persists throughout life in humans with only minor changes during ageing17. However, a note of caution should be raised in view of the fact that available animal models, in spite of recapitulating many disease features,do not replicate the entire genetics of DS.The effects of multiple genetic interactions on the treatment outcome cannot be fully controlled, hampering the translational predictivity of studies in animals7. Hence, proof-of-concept studies in DS subjects are warranted to investigate this approach. Lithium is a well-established, relatively well-tolerated mood stabiliser and is widely used in therapy of mood disorders.However,it has adverse effects that might be problematic in the DS population, prompting caution with its therapeutic application. Still, given its well-known therapeutic and safety profile, lithium might be useful for validating the neurogenesis target in DS subjects. Importantly, in the Ts65Dn mouse, the effects of lithium manifest at doses achieving plasma concentrations consistent with the human therapeutic range (0.5-1.2 mEq/L). Nonetheless, one key factor shall be taken into account to design clinical trial of pro-neurogenic treatments. In DS, changes in neurogenesis are only part of a more complex and distributed pathology. In fact, DS patients often develop Alzheimer’s disease (AD) at young age2, which is accompanied by synaptic pathology and hippocampal deficits. Therefore, eligibility criteria shall be carefully set, limiting the study population to 30-40 year-old young adults to maximise the potential efficacy of the treatment and avoid confounding effects from neurodegenerative mechanisms. Notably, lithium has been also found to reduce Beta-amyloid pathology and alleviate memory deficits both in AD transgenic mouse models and patients with mild cognitive impairment18,19. Thus, with cautious design, studies of chronic lithium administration to young DS subjects may also shed light on the potential preventive efficacy of the drug in reducing or delaying AD pathology.
In conclusion, lithium-based therapies may have important translational implications in the near future, in view of the increased prevalence of DS in EU20 and USA21 over the past decades and the increased life expectancy of DS patients due to improved medical care. Moreover, from a long-term perspective, our findings point at adult neurogenesis as a potential target to design future therapies for the treatment of selected cognitive disabilities in DS patients and other neuropsychiatric disorders with flawed adult neurogenesis.
References
- Mongiat, L. A. & Schinder, A. F. Adult neurogenesis and the plasticity of the dentate gyrus network. Eur J Neurosci 33, 1055-1061 (2011).
- Contestabile, A., Benfenati, F. & Gasparini, L. Communication breaks-Down: from neurodevelopment defects to cognitive disabilities in Down syndrome. Prog Neurobiol 91, 1-22 (2010).
- Winner, B., Kohl, Z. & Gage, F. H. Neurodegenerative disease and adult neurogenesis.Eur J Neurosci 33, 1139-1151 (2011).
- Coras, R. et al. Low proliferation and differentiation capacities of adult hippocampal stem cells correlate with memory dysfunction in humans. Brain 133, 3359-3372 (2010).
- Ming, G. L. & Song, H. Adult neurogenesis in the mammalian brain: significant answers and significant questions. Neuron 70, 687-702 (2011).
- Pennington, B. F., Moon, J., Edgin, J., Stedron, J. & Nadel, L. The neuropsychology of Down syndrome: evidence for hippocampal dysfunction. Child Dev 74, 75-93 (2003).
- Gardiner, K. J. Molecular basis of pharmacotherapies for cognition in Down syndrome.Trends Pharmacol Sci 31, 66-73 (2010).
- Reeves, R. H. et al. A mouse model for Down syndrome exhibits learning and behaviour deficits. Nat Genet 11, 177-184 (1995).
- Clark, S., Schwalbe, J., Stasko, M. R., Yarowsky, P. J. & Costa, A. C. Fluoxetine rescues deficient neurogenesis in hippocampus of the Ts65Dn mouse model for Down syndrome. Exp Neurol 200, 256-261 (2006).
- Bianchi, P., Ciani, E., Contestabile, A., Guidi, S. & Bartesaghi, R. Lithium restores neurogenesis in the subventricular zone of the Ts65Dn mouse, a model for Down syndrome. Brain Pathol 20, 106-118 (2010).
- Contestabile, A. et al. Lithium rescues synaptic plasticity and memory in Down syndrome mice. J Clin Invest 123, 348-361 (2013).
- Saxe, M. D. et al. Ablation of hippocampal neurogenesis impairs contextual fear conditioning and synaptic plasticity in the dentate gyrus. PNAS 103, 17501–17506 (2006).
- Deng, W., Aimone, J. B. & Gage, F. H. New neurons and new memories: how does adult hippocampal neurogenesis affect learning and memory? Nature reviews. Neuroscience 11, 339-350 (2010).
- Hernández-Rabaza, V. et al. Inhibition of adult hippocampal neurogenesis disrupts contextual learning but spares spatial working memory, long-term conditional rule retention and spatial reversal. Neurosci 159, 59-68 (2009).
- Clelland, C. D. et al. A Functional Role for Adult Hippocampal Neurogenesis in Spatial Pattern Separation. Science 325, 210-213 (2009).
- Garthe, A., Behr, J. & Kempermann, G. Adult-Generated Hippocampal Neurons Allow the Flexible Use of Spatially Precise Learning Strategies. Plos One 4, e5464 (2009).
- Spalding, K. L. et al. Dynamics of hippocampal neurogenesis in adult humans. Cell 153, 1219-1227 (2013).
- Zhang, X. et al. Long-term treatment with lithium alleviates memory deficits and reduces amyloid-beta production in an aged Alzheimer’s disease transgenic mouse model. J Alzheimers Dis 24, 739-749, doi:10.3233/JAD-2011-101875 (2011).
- Forlenza, O. V. et al. Disease-modifying properties of long-term lithium treatment for amnestic mild cognitive impairment: randomised controlled trial. Br J Psychiatry 198, 351- 356 (2011).
- Dolk, H. et al. Trends and geographic inequalities in the prevalence of Down syndrome in Europe, 1980-1999. Rev Epidemiol Sante Publique, 2S87-95 (2005).
- Shin, M. et al. Prevalence of Down syndrome among children and adolescents in 10 regions of the United States. Pediatrics 124, 1565-1571 (2009).