Abstract
Prior to the widespread advent of frameless stereotaxy, intracranial tumour surgery relied upon a 3-D conceptualisation of anatomy from pre-operative imaging. External anatomical landmarks such as the coronal suture and auricle helped guide the placement of a craniotomy. Bone flaps were often planned with a margin for error, particularly when locating non-lobar tumours. The choice of an appropriate trajectory for a burr hole biopsy was frequently sub-optimal with negative biopsies and significant associated morbidity. For less accessible lesions a frame-based stereotactic craniotomy was performed. This entailed placement of a stereotactic frame, acquisition of pre-operative images and calculation of tri-planar coordinates. Such procedures, although cumbersome and to some extent restrictive in the access they provide, remain part of the armamentarium for small deep targets. However, the evolution of sophisticated, accurate frameless stereotaxy has significantly changed the surgical approach to the majority of patients with space occupying lesions [1]. Application of stereotactic technology can also be used to locate other lesions such as cavernomas and abscesses. Neuronavigation is also used to facilitate rigid endoscopic procedures. This paper aims to outline the principles of neuronavigation for neuro-oncology.
System components
- Several manufacturers supply serviceable neuronavigation systems. The fundamental components include:
- Computer workstation (pre-operative and intra-operative) with neuronavigation software and computer monitor
- Medical imaging input (either through a DICOM link or a portable digital data format)
- Optical digitiser with infrared emitters and two infrared cameras
- Reference frame (secured to a head clamp)
- Registration stars (frames) for surgical instruments.
- Passive infrared reflectors (aluminium impregnated glass spheres)
Understanding the basics
- The principal concepts that underpin neuronavigation are simple:
- Pre-operative imaging. This must comprise sequential, non-overlapping volumetric slices without interspacing. Most commonly MRI slices of 1-3 mm are used to provide this ‘image dataset’.
- Accurate registration of the ‘image dataset’ with the ‘real time surgical space’.
- A triangulation based system (analogous to Satellite navigation) to track operative instruments.
- A dynamic referencing system to maintain the validity of registration during the operative procedure.
Pre-operative imaging
The mainstay of pre-operative diagnostic imaging in patients with intracranial mass lesions is the MRI scan. If each slice represents a known thickness of brain tissue (e.g. 2mm) and the slices are contiguous without overlaps or spaces, the summation of the slices creates a 3-D reconstruction of the brain. This digital imaging dataset is then transferred to the workstation. Software enables the imaging dataset to be viewed in multiple planes and as a 3-D reconstruction, permitting pre-operative planning to be undertaken (Figure 1). The target lesion is identified and the surgical approach planned. Image fusion software can now fuse digitised information from other modalities such as CT and PET with the MRI scan at this stage [2]. Although functional imaging is not yet widely available preliminary experience indicates that delineation of eloquent cortex will assist surgical planning (Figure 2). The planning software has now reached a level of sophistication whereby the tumour and other structures (e.g. cranial nerves, vessels, eloquent cortex) can be independently outlined and shaded to facilitate surgery.
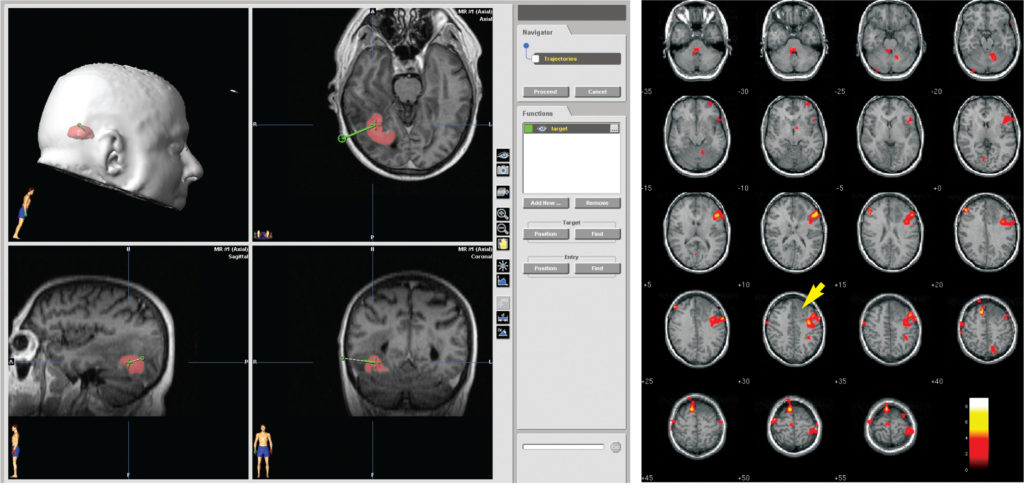
Figure 2 (right): Positron Emission Tomograph demonstrating regional cerebral blood flow in a comprehension language paradigm in a patient with a low grade glioma. Note the proximity of the speech cortex to the tumour. The incorporation of functional information into the navigation system is of immense potential benefit.
Registration
Registration is the process by which the pre-operative image dataset is aligned with the real-time anatomy of the surgical space in the operating theatre (Figure 3) [3]. To prevent movement the patient’s head is held in a Mayfield Clamp in view of the Optical Digitiser. Registration is then performed using either a point or surface alignment technique. The workstation/optical digitiser recognises a default surgical pointer instrument within the field of view. The tip of this tool is then used to identify the real-time location of widely spaced, non-collinear fiducials (surface markers) placed on the surface of the head prior to the pre-operative image dataset acquisition. The co-alignment of these image dataset points with the surgical field achieves registration. Alternatively, anatomical landmarks identifiable on the imaging and in the surgical field (e.g. nasion, external auditory canal, orbital margins) can be co-aligned for registration. Registration using surface alignment uses complex mathematical algorithms to co-align the surface of the patient’s head in the operating theatre with the surface of the image dataset. A Laser beam is used within sight of the optical digitiser to delineate the peri-orbital and forehead regions of the scalp. Co-alignment of the thousands of surface points identified by the Laser and the surface contours of the image dataset enables registration. The manual addition of another 10 or so points from all quadrants of the scalp using the default pointer further refines the accuracy of this technique.
The accuracy of registration must be confirmed with visual checks. The computer monitor shows the pre-operative image dataset (usually in axial, coronal and sagittal planes with a 3D reconstruction view). Known landmarks on this dataset are identified in the surgical space using the default pointer tool (e.g. globes, orbits, external auditory meatus, tragus). The radiological and real-time anatomical landmarks should coincide exactly. If there are discrepancies the registration process should be repeated.
Triangulation based instrument tracking
If 3 or 4 passive infrared reflectors are clamped via a star-shaped reference frame to a surgical instrument, and the tip of the instrument is registered with a known location, intraoperative tracking can be performed. A triangulation principle is used to achieve this aim. Infrared light emitted by the optical digitiser is reflected from the passive reflectors to the 2 cameras. The position of the instrument can then be calculated and transcribed onto the imaging dataset enabling intraoperative tracking on the navigation system monitor.
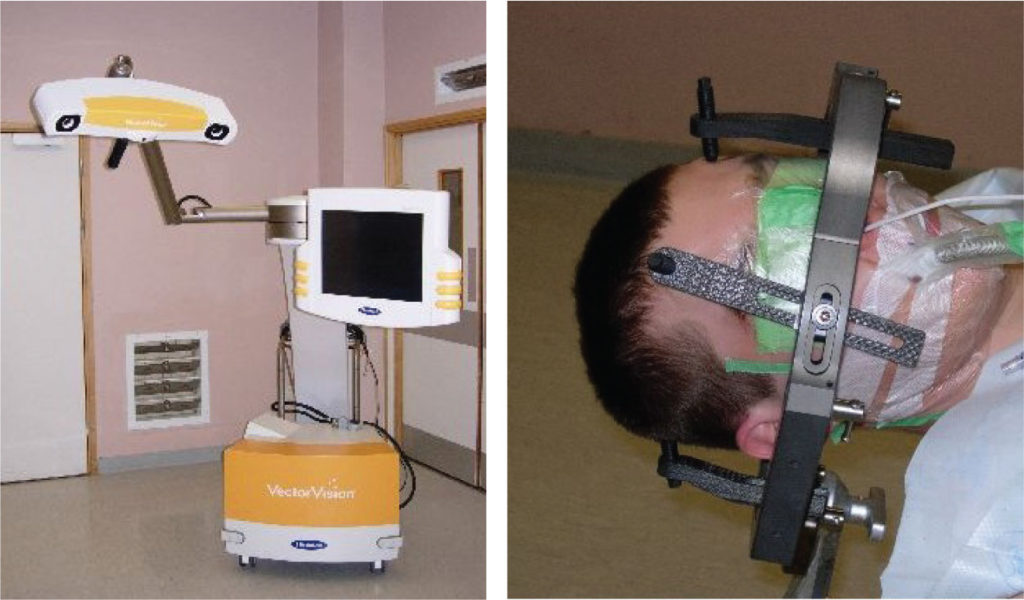
Figure 4 (right): This patient had a small cavernoma just beneath the motor strip. The lesion could not be located with frameless stereotaxy. Image fusion utilising MRI and CT scans was performed to maximise the quality of the imaging dataset prior to a frame-based stereotactic procedure. This technique enabled accurate identification of the lesion that was resected without incurring any neurological deficit.
Dynamic referencing
Microsurgical approaches demand a straight corridor of access to permit illumination of the target area. To facilitate access and to provide manoeuvrability around the circumference of a tumour, frequent adjustments in operating table position are necessary during a resection procedure. Each movement alters the spatial relationship between the real-time surgical space and the previously registered position of the imaging dataset. To prevent such adjustments from invalidating registration, an optical reference frame with passive infrared reflectors is secured to the Mayfield head clamp. The optical digitisers recognise the fixed relationship between the reference frame and the patient’s head. Any movement of the operating table therefore moves not only the patient but also the dynamic reference frame, maintaining valid registration. Similarly, if the optical digitiser is moved, the constant spatial relationship between the reference frame and the surgical space maintains registration.
Intra-operative navigation
Craniotomy
Following registration, neuronavigation can be performed. Initially the pointer can be used to map out the site of a craniotomy flap. For small convexity meningiomas the bone flap can be accurately sited minimising the size of the flap. Care needs to be exercised when drawing a flap to account for parallax-type errors. These are minimised by planning a bone flap perpendicular, rather than oblique, to the bone. When working intracranially it is convenient to register familiar surgical instruments that can then be tracked as described above. The operating microscope can also be used as a surgical tool. The microscope has a passive reflector star secured in the line of sight from the optical digitiser. This permits the focal point of the microscope to be tracked in the surgical space and observed in the image space. The previously outlined target lesion (from pre-operative planning) can also be visualised in a “head-up” display to help direct both the dissection to the target and to guide resection margins during the procedure.
Burrhole Biopsy
Neuronavigation can be used to perform biopsies with minimal trauma to the brain. A lockable trajectory arm is secured to the Mayfield clamp. A 2.0mm drill guide with a registration star attached is then used in conjunction with a concentric ring target view that enables the required trajectory to be determined. The addition of a virtual tip extension to the drill guide confirms that the target is at the centre of the trajectory. The drill guide is then secured in the lockable trajectory arm and a small twist drill hole performed through the guide. After opening the dura and keeping the position of the trajectory arm constant a disposable double lumen side cutting sedan needle is inserted through the drill guide to perform the biopsy at the appropriate depth. Registration of the needle can be undertaken to allow real-time visualisation of the biopsy on the pre-operative image dataset. The outer sheath of the needle remains in situ whilst samples are taken at 12, 4 and 8 o’clock. The needle can then be withdrawn a few millimetres to permit repeat sampling, minimising sampling errors.
Neuronavigation errors
Errors can occur at any stage during the neuronavigation process. These can seriously compromise the accuracy of neuronavigation. Diligence therefore needs to be observed at all stages to minimise, recognise and avoid errors (Figure 4). Some of these are shown in Table 1. The use of intraoperative MRI scanning with all its associated inherent problems aims to recognise brainshift and enable an up to date imaging dataset to be used. Whilst time consuming and expensive such technology may have a role to play, particularly during resective procedures for low-grade gliomas.
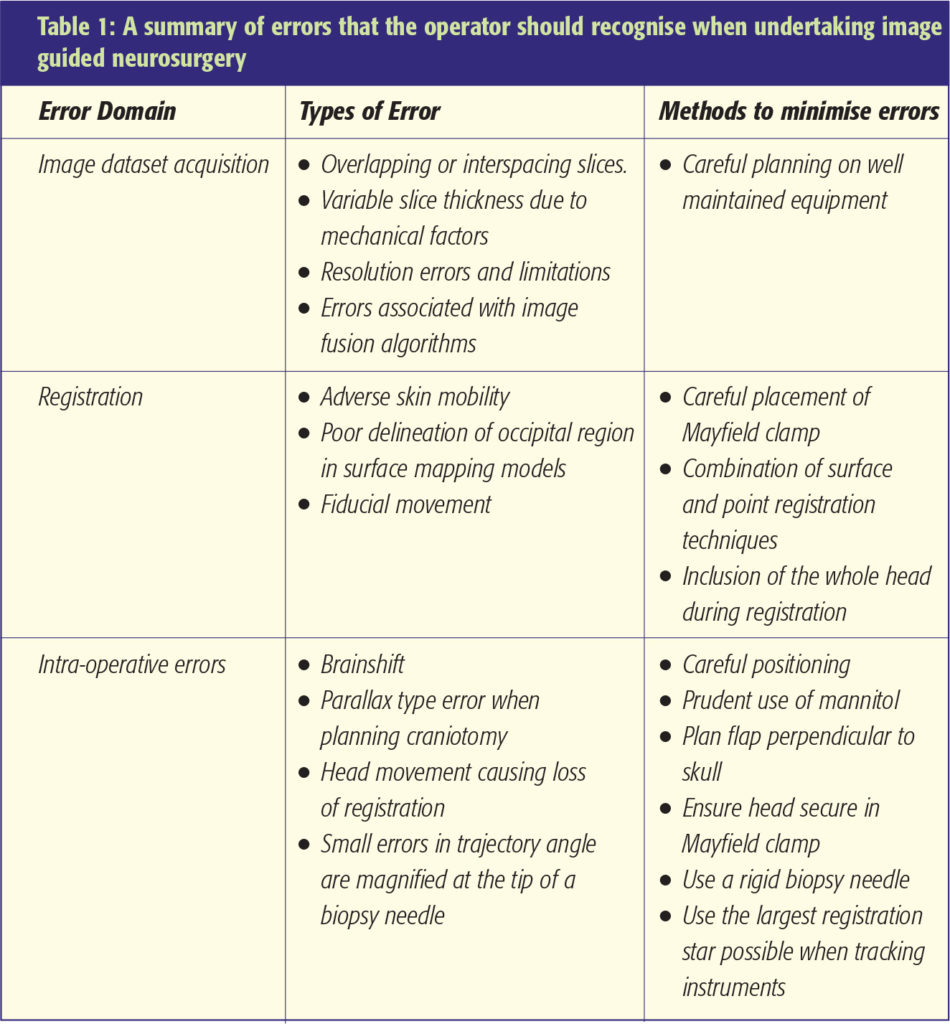
Summary
The rapid evolution of neuronavigation over the past decade has led to numerous publications describing the application and advancement of such technology. In this era of evidence-based medicine most of the literature supporting the use of neuronavigation comprises single centre case series. However, the confidence instilled by this technology in the surgical fraternity is such that randomised trials of efficacy are considered by most as inappropriate and unethical. Future developments are likely to be targeted at circumventing neuronavigation errors and providing up to date image sets during surgery.
References
- Watanabe E, Mayanagi Y, Kosugi Y, Manaka S, Takakura K. Open surgery assisted by the neuronavigator, a stereotactic, articulated, sensitive arm. Neurosurgery 1991;28:792-9. https://doi.org/10.1227/00006123-199106000-00002
- Schulder M, Maldjian JA, Liu WC, Holodny AI, Kalnin AT, Mun IK, Carmel PW. Functional image-guided surgery of intracranial tumors located in or near the sensorimotor cortex. Journal of Neurosurgery 1998;89(3):412-8. https://doi.org/10.3171/jns.1998.89.3.0412
- Bucholz R, Marzouk S, Levy A. Image guidance and the operating microscope: Stealth and neural navigation. Chapter 28 in Advanced Neurosurgical Navigation Eds. E. Alexander III, RJ Maciunas (pp345-55). Thieme Medical Publishers Inc. New York 1999.