Low grade glioma is an uncommon diagnosis. Patients are younger than those with high grade glioma and most commonly present with seizures. The diagnostic modality of choice is MRI. There is a significant risk of dedifferentiation of residual LGG to higher grade secondary to accumulation of genetic alterations. Whilst many patients in low risk prognostic groups remain stable for years, the median survival is in the region of ten years. Clinical, radiological and pathological prognostic factors have been identified. Management is carried out under the auspices of an MDT. Surgery currently remains the mainstay of treatment with a trend to aim for gross total resection in suitable patients. Surveillance alone or biopsy and subsequent surveillance have a role to play in some cases. Various adjuncts are available, but radiotherapy may be best reserved for high risk patients or until there is evidence of tumour progression. Future advances may eventually be able to justify the “benign” nature implied by the term “low-grade” glioma.
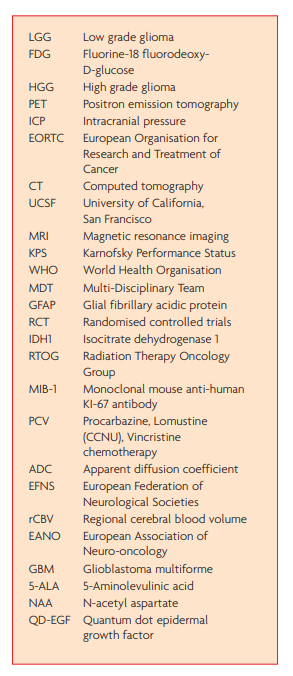
Introduction
The first reported glioma resection was performed in London, by Sir Rickham Godlee, in 1884, after a physician colleague, Dr Alexander Hughes Bennett, correctly surmised that a 25-year old man with seizures and progressive left hemiparesis must have a right-sided cortical tumour [1-3]. Although primary central nervous system neoplasms represent 2% of all cancers, intrinsic low grade gliomas (LGG) are uncommon, representing 15% of this total. The incidence is about 1 in 100,000 per year and they present at a younger age than high grade gliomas, most commonly at age 30-40 [1,4,5]. Like the higher grade gliomas (HGG), there is a slightly increased incidence in males.
Presentation
80% of LGG typically present with seizures [1,5,6]. Frequently these can be resistant to drug therapy [1,5]. Increased availability of imaging has resulted in more “incidental” findings. Although patients appear neurologically intact, neuropsychology assessments reveal abnormalities in 91% of patients [7]. These neurocognitive deficits affect quality of life. Large lesions may present with symptoms of raised intracranial pressure (ICP) or focal neurological deficit, though new presentation of these symptoms in a previously known lesion may be a harbinger of progression and/or transformation.
Investigation
Conventionally these lesions have been diagnosed and investigated using computed tomography (CT) and magnetic resonance Imaging (MRI). CT demonstrates an area of hypodensity, but tumours may be difficult to detect or substantially larger than visualised, often being near iso-dense. Calcification may be seen in 20% of diffuse astrocytomas and 40% of oligodendrogliomas. On MRI, low-grade gliomas usually appear as low signal on T1 weighted imaging and a well-defined area of high signal on T2, often with a more extensive abnormality than seen on CT. See Figure 1. FLAIR sequences attenuate the signal from CSF and can better define peri-ventricular lesions. Typically, in contrast to high grade gliomas, there is little mass effect and little surrounding vasogenic oedema. The specificity and sensitivity of contrast enhancement, as an indication of high tumour grade, is poor: 20-60% of grade I-II oligodendrogliomas enhance. Conversely, anaplastic astrocytomas (WHO grade III lesions) fail to enhance in 31-54% of cases [8], as do even 4% of glioblastomas (WHO grade IV) [1]. Thus, conventional imaging has substantial limitations.
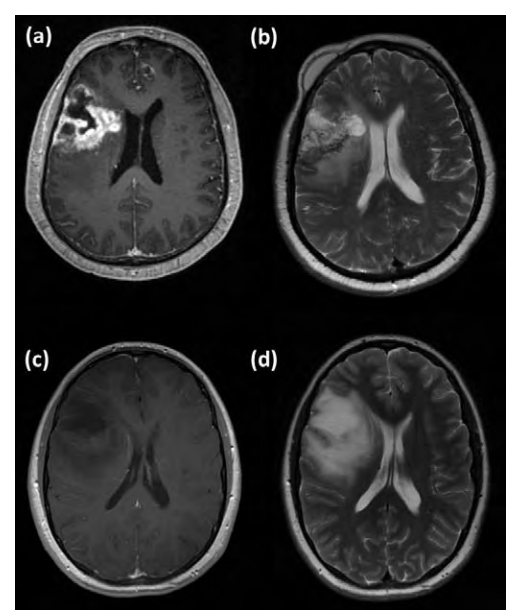
Histology
Low grade gliomas arise from supporting glial cells in the brain. The predominant cell type determines the pathological classification. Tumours are graded according to the World Health Organisation (WHO) grading system (grades I to IV; grades III and IV are considered malignant) [4]. LGG are a heterogenous group of WHO grade I and II tumours. The most common histological subtypes are pilocytic astrocytomas (WHO gd I), ependymomas and the diffuse gliomas: astrocytomas (gd II), oligoastrocytomas (gd II) and oligodendrogliomas (gd II). Here we discuss low grade diffuse gliomas. See Figure 2. The tumours have low cellularity and normal brain tissue is mixed in with the tumour [5]. Anaplasia and mitoses are absent (though a single mitosis is allowed), vascularity is lower than in high grade tumours. Necrosis is absent [4]. Histological stains mark for cell type and proliferation. GFAP (glial fibrillary acidic protein) and S-100 protein are both found in the central nervous system and can be markers that the tumour is of glial origin: S100 is less specific and is also found in Schwann cells, chondrocytes and melanomas. Conversely cytokeratin is found in epithelial cells and therefore stains metastatic carcinoma, but not primary glioma. Staining for IDH1 mutation is positive in 70-80% LGG, but rare in HGG. MIB-1 (monoclonal mouse anti-human KI-67 antibody) stains cells leaving the G0/G1 phase of the cell cycle i.e. performing DNA synthesis. A high MIB-1 index indicates high mitotic activity and correlates with malignancy [9 ]. Genetic studies have demonstrated patterns of mutation: p53 and 17p mutations are associated with diffuse astrocytoma, whereas loss of the short arm of chromosome 1 is found in oligodendroglioma [10,11].
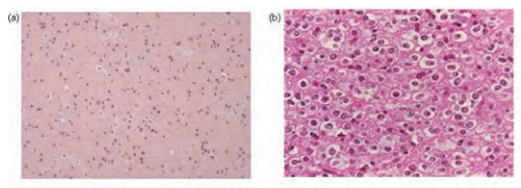
Modern imaging
Modern imaging techniques can take advantage of the histological features to differentiate low-grade gliomas from high grade tumours. Because of their low cellularity, LGG have a higher apparent diffusion coefficient (ADC) on diffusion weighted MRI than HGG. HGG have a higher regional blood flow (rCBV) than LGG. See Figure 3. Perfusion studies can reliably differentiate grade II diffuse astrocytoma from glioblastoma multiforme (GBM), but discriminating between grade II versus III and III versus IV is more difficult because of marked overlap in appearances between individual tumours. Perfusion imaging may also distinguish radiation-necrosis (low rCBV) from tumour recurrence (high rCBV) [8].
Metabolism and proliferation are elevated in HGG, but normal or lower than normal brain in LGG. MR spectroscopy non-invasively assesses the metabolism of a small region (single voxel) of brain. In all gliomas, the choline peak (a marker of membrane turnover) is increased and N-acetyl aspartate (NAA, a neuronal marker) is reduced. Lipid, marking necrosis, and lactate, marking hypoxia, are increased in HGG, but not in LGG. See Figure 4. MR spectroscopy may also be useful in differentiating cortical dysplasia and other benign conditions from LGG. Fluorine-18 fluorodeoxy-D-glucose (FDG) labelled positron emission tomography (PET) imaging quantifies glucose utilisation, which is increased in HGG but the same as, or lower than, normal brain in LGG. Again, it can also be useful to differentiate tumour recurrence (hyper metabolic) from radio-necrosis (hypo-metabolic). FDG PET is, however, limited by the uptake of FDG in all metabolically active areas of the brain. Methionine PET provides a measure of increased protein synthesis due to incorporation of this into proteins. It appears to be a more reliable marker of proliferating tissue than FDG PET and can distinguish between neoplastic and inflammatory processes. FLT (a thymidine analogue) marks DNA synthesis and correlates with MIB-1 index on histological staining. However, FLT can give false positives in LGG after radiotherapy due to disruption of the blood brain barrier [8].
Modern imaging techniques thus offer additional information to that provided by conventional imaging. Whilst not yet approaching the gold-standard of tissue histology, they may optimise target selection for diagnostic biopsy [8].
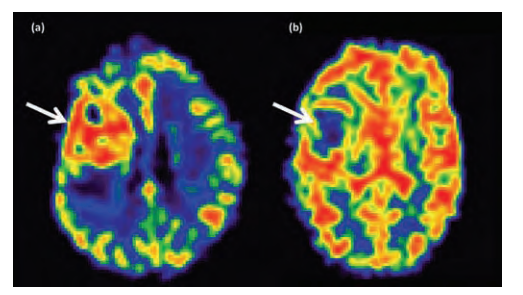
Prognosis
The ultimate behaviour of these tumours is not benign. The natural history is of serial accumulation of genetic abnormalities resulting in malignant transformation and, ultimately, death [1]. The behaviour of LGG is highly variable and whilst some may remain stable for a long period, others dedifferentiate sooner [12]. In studies pre-1990, a 5-year survival rate of 40-50% was reported [1]. More recently, data show a median-survival approaching 10 years. Whether this advantage is entirely due to improved treatment, or whether this is in part due to ‘starting the clock earlier’ with earlier diagnosis from better imaging modalities, is unclear [1].
Many studies have undertaken a multivariate analysis to evaluate potential prognostic factors. A number of patient-related, tumour-related and treatment-related prognostic factors have been elucidated [12]. Young age, good performance status and presentation with seizures confer a survival advantage. Symptoms of raised ICP or focal neurological deficit are associated with a worse prognosis. Small tumours and oligodendroglioma subtype are positive prognostic factors. Larger tumours (greater than four-six centimetres) [12-14] poorly circumscribed tumours or multifocal lesions have a worse prognosis. Pilocytic astrocytoma is WHO grade I and may be cured by gross total resection. Gemistocytic subtype tumours have a high risk of anaplastic transformation. The 1p19q chromosome deletion correlates with oligodendroglioma subtype and is a marker of good prognosis [1,4,13,15]. The p53 gene mutation (more common in astrocytomas) [13] and a high MIB-1 proliferation index (>8%) on histological staining [5] carry a poorer prognosis. More recent studies have shown that the IDH1 mutation is a common, early mutation in both astrocytic and oligodendroglial tumours that predicts prolonged survival [16]. Growth rate predicts prognosis [17,18]. Evidence from imaging studies shows that these tumours grow with a mean increase in diameter of 4mm/year. Tumours growing faster than 8.1mm/year in diameter have a median survival of just five years versus over 15 years for those growing more slowly [17]. Recent imaging studies have shown that rCBV is also an important marker of prognosis. Patients with regions of increased rCBV have a much lower overall survival [19,20]. The increase in rCBV can be seen up to six months before these tumours clinically transform [20]. Further studies are required to see if this could predict when to initiate further treatment.
Prognostic scores have been developed based on these factors. See Table 1. These high- versus low-risk groups may be useful to guide management strategies.
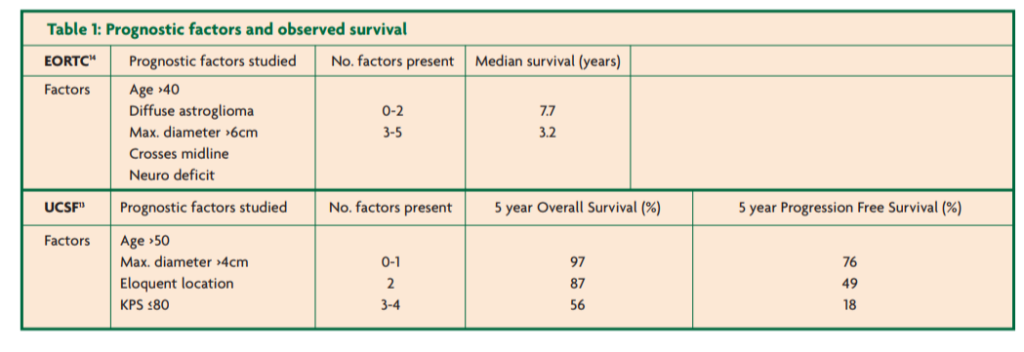
Management
The management of LGGs should be conducted through a multi-disciplinary team (MDT) approach [21]: options include conservative and operative approaches.
Conservative management
Conservative management may be initiated at a number of stages. A “watch and wait” policy may be followed from the outset with radiological surveillance, without histological diagnosis. These patients would have imaging demonstrating lesions without any features associated with malignancy and would be stratified in a low-risk group [5,15]. They may have a long, stable history of well-controlled seizures or have a deep lesion not amenable to biopsy. Informed patient-preference must play a role. However, in the absence of a pathognomonic radiological appearance [13] of “low-gradeness”, and the fact that up to 20% of tumours thought to be grade II radiologically are actually grade III histologically [5], a conservative approach requires careful consideration. Conservative management may also be
followed after diagnostic stereotactic biopsy [22].
Surgery
Surgery ranges from diagnostic biopsy, to debulking, to gross total resection. Stereotactic biopsy is minimally invasive, well-tolerated and suitable for deep lesions. Larger lesions may be biopsied using frameless image guidance. Biopsy has the limitations of small sample size which can fall foul of the heterogenous nature of LGG resulting in under-grading of a more aggressive tumour or producing a sampling error in mixed oligoastrocytoma (which would be more suitable for early adjunctive chemotherapy than pure astrocytoma) [5,13].
Intuitively, cytoreductive surgery, i.e. debulking, has the benefit of reducing the tumour load, perhaps reducing the risk of future disease progression. In the absence of level one studies (randomised controlled trials), many authors claim that the weight of evidence is in favour of attempted resection in suitable cases with reduced rates of recurrence and transformation and improved survival [13,23,26]. One of the major difficulties in interpreting the studies looking at the extent of resection is that they rarely report objective measurements achieved by comparing preand post-operative imaging. In addition, other authors note that the groups undergoing gross total resection, debulking and biopsy of unresectable lesions are not directly comparable. Those most suitable for gross total resection may already have a better prognosis [1,5].
The development of a range of surgical adjuncts (pre-operative PET, functional MRI and Wada test; operative tools such as the ultrasonic aspirator, operating microscope, stereotactic methods, intraoperative ultrasound, intraoperative stimulation and awake craniotomy) has been associated with an improvement in the morbidity and mortality profile of surgery. Intra-operative MRI is a modern tool to maximise resection but is not yet widely available [27]. Functional mapping may increase extent of resection and improve overall survival [26]. The rate of significant morbidity in debulking is now around 2% [28]. Whilst pre-existing neurological deficits may be temporarily exacerbated, most patients return to baseline or better within three months [13]. Debulking can provide immediate benefit in relief of mass effect and raised intracranial pressure. It can improve deficits which are due to the mass effect of the tumour (rather than those due to direct invasion) [5,28]. It allows reduction in steroid use if post-operative radiotherapy is required. It may also give better diagnostic information from the larger histological sample. In LGG with medically intractable seizures, resection improves seizure control [1].
Radiotherapy
The role of radiotherapy after biopsy or debulking is debated. An EORTC randomised controlled trial (RCT) reported longer (radiological) progression free survival (5.3 years versus 4 years) and better seizure control for the group of LGG patients treated with early radiotherapy than those given delayed treatment at the time of progression, but found no difference in overall survival. However, over one third of patients in the conservative group did not require radiotherapy during the follow up period [29]. Two prospective RCTs found no difference in outcome between a high-dose radiotherapy group and a lower-dose, but the high-dose group had a poorer quality of life [15,30]. Dose-related radiological abnormalities included white matter changes and radionecrosis. Such radiation leukoencephalopathy can result in gait disturbance, urinary incontinence, deterioration in personality and cognitive dysfunction [13]. Patients treated with high dose radiotherapy reported poorer quality of life,especially fatigue, insomnia and emotional symptoms [15]. Medium-term follow up (six years) of one cohort had suggested that radiotherapy only caused cognitive side effects at higher doses. However,more recent data show that long term survivors (12-year follow-up) who have had even lower-dose radiotherapy develop progressive decline in attention, executive functioning and information processing. Those who did not have radiotherapy had stable cognitive status, with 27% having a cognitive deficit (which was attributed to their tumour and possibly influenced by antiepileptic use) compared to 53% of those who had undergone radiotherapy [31]. Thus, given a prognosis in excess of 10 years in low risk groups, the decision whether or not to give early radiotherapy must be informed by the potential benefits in symptom control, including better seizure regulation, and the risk of long-term side effects. Keeping radiotherapy in reserve for use at the time of disease progression may be the optimal strategy.
Chemotherapy
Chemotherapy is not in routine use for astrocytic LGG but is known to be effective for oligodendroglioma subtypes (grade II and III) and for HGG. Early data from the Radiation Therapy Oncology Group (RTOG) did not show any advantage to adding PCV chemotherapy to radiotherapy for grade II tumour patients deemed at high risk [15]. Phase II trials demonstrate that temozolamide is active against both untreated and previously irradiated enlarging LGGs. In addition, there are reports of cases where neoadjuvant chemotherapy given to surgically irresectable tumours has allowed subsequent gross total resection [13,15]. The role of chemotherapy is to be investigated in the BR13 (EORTC 22033-26033) trial (www.eortc.be/protoc/details.asp?protocol=22033).
The future
A century and a bit on, modern neurooncology would be unrecognisable to Sir Rickham Godlee.MRI has improved pre-operative specificity of diagnosis: additional technologies such as PET are used to target treatment in difficult cases. Image-guided stereotactic techniques have become established in the last two decades, enabling mini-craniotomies to be placed directly over brain tumours, whilst maximising resections with reduced morbidity. The safety and accuracy of deep brain tumour biopsies has been optimised.The morbidity and mortality of surgery has been reduced from 50-100% – “…many ‘successful’ operations resulted in dead patients”2 – to 2% [22].
Progress continues. Recent evidence-based guidelines published by the European Federation of Neurological Societies (EFNS) and the European Association of Neurooncology (EANO) [32] should help to develop services for patients with low grade gliomas. Non-invasive investigations are likely to better inform the diagnostic process. A number of molecular markers (from biopsy tissue and from blood) are under investigation for utility in diagnosis and prognostication [13,33]. Newer imaging techniques continue to improve and in the future may have the potential to avoid biopsy altogether [8]. Operative techniques such as pre-operative functional mapping, intraoperative MRI and other imaging modalities are likely to become more widely used. Fluorescent markers such as 5-aminolevulinic acid (5-ALA) are proving useful in improving extent of resection in high grade glioma [34], but cannot detect LGG [35]. In vitro studies of new markers, such as quantum dot epidermal growth factor (QD-EGF), demonstrate potential for the development of similar tools for LGG [36].
Whilst radiotherapy is often reserved for progressive disease, newer highly conformational techniques may improve outcomes. Modern radiotherapy techniques can significantly reduce the radiation dose to normal brain, but are limited by the difficulty in identifying the tumour margin and by tumour volume [15,30]. Further imaging developments are needed to better delineate these tumours.
The role of concurrent chemotherapy in astrocytic LGG remains unclear. The European Organisation for Research and Treatment of Cancer (EORTC) and the Radiation Therapy Oncology Group (RTOG) are conducting clinical studies that aim to assess the value of radiation plus concurrent chemotherapy in high risk LGG patients [13,33].
References
- Kaye AH and Laws ER. (2001). Brain Tumors. 2nd Ed. Churchill Livingstone.
- Bliss M. (2005). Harvey Cushing: A life in surgery. pp169-172. Oxford University Press. https://doi.org/10.1093/oso/9780195169898.001.0001
- Pereira E, Green A, Cadoux-Hudson T. Neurosurgery through the looking glass. ACNR. 2010;10(1):32-6.
- du Plessis D. Primary brain tumours. ACNR. 2005;4(6):17-19.
- Batjer HH and Loftus CM. (2003). Textbook of neurological surgery. Principles and practice: Volume two. Ch.102:1257-1270. Lippincott, Williams & Wilkins.
- Chang EF, Potts MB, Keles GE, Lamborn KR, Chang SM, Barbaro NM, Berger MS. Seizure characteristics and control following resection in 332 patients with lowgrade gliomas. J Neurosurg. 2008;108(2):227-35. https://doi.org/10.3171/JNS/2008/108/2/0227
- Tucha O, Smely C, Preier M, Lange KW. Cognitive deficits before treatment among patients with brain tumors. Neurosurgery. 2000;47(2):324-34. https://doi.org/10.1097/00006123-200008000-00011
- Price SJ. Advances in imaging low grade gliomas. Advances and Technical Standards in Neurosurgery. 2009;35:1-34.
- Russell DS and Rubenstein LJ. (1989). Pathology of tumours of the nervous system. Williams & Wilkins.
- Barbashina V, Salazar P, Holland EC, Rosenblum MK, Ladanyi M. Allelic losses at 1p36 and 19q13 in gliomas: correlation with histologic classification, definition of a 150-kb minimal deleted region on 1p36, and evaluation of CAMTA1 as a candidate tumor suppressor gene. Clin Cancer Res. 2005;11(3):1119-28.
- Walker C, Haylock B, Husband D, Joyce KA, Fildes D, Jenkinson MD, Smith T, Broome J, du Plessis DG, Warnke PC. Clinical use of genotype to predict chemosensitivity in oligodendroglial tumors. Neurology. 2006;66(11):1661-7.
- Stupp R, Janzer RC, Hegi ME, Villemure JG, Mirimanoff RO. Prognostic factors for low-grade gliomas. Semin Oncology. 2003;30(6 S19):23-8. https://doi.org/10.1016/S0093-7754(03)00305-1
- Pouratian N and Schiff D. Management of low-grade glioma. Curr Neurol Neurosci Rep. 2010;10(3):224-31. https://doi.org/10.1007/s11910-010-0105-7
- Pignatti F, van den Bent M, Curran et al. Prognostic factors for survival in adult patients with cerebral lowgrade glioma. J Clin Oncol, 2002;20:2076-84. https://doi.org/10.1200/JCO.2002.08.121
- Baumert BG and Stupp R. Low-grade glioma: a challenge in therapeutic options: the role of radiotherapy. Annals of Oncology. 2008;19(S7):vii217-vii222. https://doi.org/10.1093/annonc/mdn434
- Dubbink HJ, Taal W, van Marion R, Kros JM, van Heuvel I, Bromberg JE, Zonnenberg BA, Zonnenberg CB, Postma TJ, Gijtenbeek JM, Boogerd W, Groenendijk FH, Smitt PA, Dinjens WN, van den Bent MJ. IDH1 mutations in low-grade astrocytomas predict survival but not response to temozolomide. Neurology. 2009;73(21):1792-5. https://doi.org/10.1212/WNL.0b013e3181c34ace
- Mandonnet E, Delatte JY, Tanguy ML, Swanson KR, Carpentier AF, Duffau H et al. Continuous growth of mean tumor diameter in a subset of grade II gliomas. Ann Neurol. 2003;53(4):524-8. https://doi.org/10.1641/0006-3568(2003)053%5B0524:EEEBBA%5D2.0.CO;2
- Pallud J, Mandonnet E, Duffau H, Kujas M, Guillevin R, Galanaud D, Taillandier L, Capelle L. Prognostic value of initial magnetic resonance imaging growth rates for World Health Organization grade II gliomas. Ann Neurol. 2006;60(3):380-3.
- Law M, Oh S, Babb JS, Wang E, Inglese M, Zagzag D, Knopp EA, Johnson G. Low-grade gliomas: dynamic susceptibility-weighted contrast-enhanced perfusion MR imaging–prediction of patient clinical response. Radiology. 2006;238(2):658-67. https://doi.org/10.1148/radiol.2382042180
- Danchaivijitr N, Waldman AD, Tozer DJ, Benton CE, Brasil Caseiras G, Tofts PS, Rees JH, Jäger HR. Low-grade gliomas: do changes in rCBV measurements at longitudinal perfusion-weighted MR imaging predict malignant transformation? Radiology, 2008;247(1):170-8. https://doi.org/10.1148/radiol.2471062089
- National Institute for Health and Clinical Excellence. Improving Outcomes for People with Brain and Other CNS Tumours. 2006. Downloaded from http://www.nice.org.uk/nicemedia/live/10905/28963/28 963.pdf
- Whittle IR. What is the place of conservative management for adult supratentorial low-grade glioma? Adv Tech Stand Neurosurg. 2010;35:65-79. https://doi.org/10.1007/s00355-009-0430-4
- Keles GE, Lambrorn KR, Berger MS. Low-grade hemispheric gliomas in adults: a critical review of extent of resection as a factor influencing outcome. J Neurosurg. 2001;95(5):735-45.
- Smith JS, Chang EF, Lambourn KR et al. Role of extent of resection in the long-term outcome of low-grade hemispheric gliomas. J Clin Oncol. 2008;26:1338-45. https://doi.org/10.1200/JCO.2007.13.9337
- McGirt MJ, Chaichana KL, Attenello FJ et al. Extent of surgical resection is independently associated with survival in patients with hemispheric infiltrating lowgrade gliomas. Neurosurgery. 2008;63:700-7. https://doi.org/10.1227/01.NEU.0000325729.41085.73
- Berger MS. Defining and achieving excellence in surgical neuro-oncology. Clinical Neurosurgery. 2010;57:10-14.
- Hatiboglu MA, Weinberg JS, Suki D, Rao G, Prabhu SS, Shah K, et al. Impact of intraoperative high-field magnetic resonance imaging guidance on glioma surgery: a prospective volumetric analysis. Neurosurgery. 2009;64(6):1073-81.
- Duffau H. Surgery of low-grade gliomas: towards a ‘functional neurooncology’. Curr Opin Oncol. 2009;21(6):543-9.
- van den Bent MJ, Afra D, de Witte O et al. EORTC Radiotherapy and Brain Tumour Groups and the UK Medical Research Council. Long-term efficacy of early versus delayed radiotherapy for low-grade astrocytoma and oligodendroglioma in adults: the EORTC 22845 randomised trial. Lancet. 2005;366:985-90. https://doi.org/10.1016/S0140-6736(05)67070-5
- Baumert BG and Stupp R. Is there a place for radiotherapy in low-grade gliomas? Adv Tech Stand Neurosurg. 2010;35:159-82. https://doi.org/10.3917/idee.159.0035
- Douw L, Klein M, Fagel SSAA, van den Heuvel J,Taphoorn MJB, Aaronson NK, Postma TJ, Vandertop WP, Mooij JJ, Boerman RH, Beute GN, Sluimer JD, Slotman BJ, Reijneveld JC, Heimans JJ. Cognitive and radiological effects of radiotherapy in patients with lowgrade glioma: long-term follow-up. Lancet Neurology. 2009;8:810-18.
- Soffietti R, Baumert BG, Bello L, von Deimling A, Duffau H, Frénay M, Grisold W, Grant R, Graus F, Hoang-Xuan K, Klein M, Melin B, Rees J, Siegal T, Smits A, Stupp R, Wick W; European Federation of Neurological Societies. Guidelines on management of low-grade gliomas: report of an EFNS-EANO Task Force. Eur J Neurol. 010;17(9):1124-33.
- Ruiz J and Lesser GJ. Low-grade gliomas. Current Treatment Options in Oncology. 2009;10:231-42. https://doi.org/10.1007/s11864-009-0096-2
- Nabavi A, Thurm H, Zountsas B, Pietsch T, Lanfermann H, Pichlmeier U, et al. Five-aminolevulinic acid for fluorescence-guided resection of recurrent malignant gliomas:a phase ii study. Neurosurgery. 2009;65(6):1070-7. https://doi.org/10.1227/01.NEU.0000360128.03597.C7
- Langen K, Ewelt C, Floeth F, Stoffels G, Felsberg J, Steiger H, Sabel M, Herzog H, Coenen H, Strummer W. Comparison of FET-PET and 5-ALA fluorescence in gliomas. J Nucl Med. 2010;51(S2):1805. https://doi.org/10.2967/jnumed.110.077982
- Kantelhardt SR, Caarls W, de Vries AHB, Hagen GM, Jovin TM, Schulz-Schaeffer W, Rohde V, Giese A, ArndtJovin DJ. Specific visualization of glioma cells in living low-grade tumor tissue. PLoS ONE. 2010;5(6):e11323. https://doi.org/10.1371/journal.pone.0011323