Freezing of gait (FOG) is a devastating symptom that affects the majority of patients with advanced Parkinson’s disease (PD). It typically manifests as a sudden inability to move the lower limbs despite the intention to walk.1 However, the phenomenon is not limited to gait and individuals with PD have been shown to suffer from freezing in other movements, such as handwriting, brushing teeth and even speech. The pathophysiology underlying freezing is not well understood but a number of recent research approaches have led to insights that offer the prospect of new avenues for therapeutic intervention and management.
Due to the obvious problems associated with the neuroimaging of gait disorders, the investigation of the neural basis of FOG has been problematic. Although potentially very informative, studies usually suffer from limits in temporal precision (such as Positron Emission Tomography) or are required to provide challenging links between imaging results and behaviour (such as tasks that measure brain activity while subjects watch a first-person perspective video of an actor walking). To combat these issues, a novel Virtual Reality (VR) paradigm in which subjects navigate a non-immersive, yet realistic three-dimensional environment using footpedals to control their ‘walking’ has been developed.2 The VR task requires bipedal motor activity whilst processing cognitive and environmental information. Importantly, the task can successfully and safely elicit motor freezing in susceptible individuals and performance on the VR task has been correlated with self-reported FOG symptoms2 and more recently, with the severity of actual recorded episodes of FOG.3
This validated VR technology can also be combined safely with fMRI to provide unique insights into the pathophysiological mechanism of freezing. A recently published study has provided evidence that freezing behaviour in the VR task is associated with hyper-activity within cortical regions subserving cognitive operations but with concomitant decreases in the activity within the striatum, a key region within the basal ganglia nuclei.4 A subsequent study has demonstrated that these cortical and subcortical regions show functional decoupling at the network level.5 That is, the cortical and subcortical structures communicate well during effective navigation of the VR task, however when a subject shows evidence of a breakdown in the normal footstep pattern, their corticostriatal networks show a similar breakdown in effective communication. Together, these imaging studies provide convincing evidence that the dynamic process of freezing is due to paroxysmal breakdowns in communication between large-scale networks within the brain.
The results from these fMRI studies are also supported by recent evidence obtained from an ambulatory electroencephalography (EEG) study in which the electrophysiological correlates of brain activity have been measured whilst patients with FOG navigated a standardised clinical walking assessment (timed up-and-go). After strict correction for movement-related artefacts, it has been shown that episodes of freezing were associated with an increase in theta band power (~3-7Hz) over the central and frontal regions, which broadly map to motor execution and planning regions of the brain, respectively. In addition, there was a transition in the degree of synchrony between different regions in the theta frequency over the temporal evolution of a freezing episode, further supporting the notion that FOG is due to impaired functional coupling between distant brain regions.
Using these objective insights has led to a revised framework6 that extends a previously proposed model of freezing behaviour,7 in which freezing behaviour was conceptualised as occurring secondary to transient increases in GABAergic neuronal activity within the output structures of the basal ganglia (such as the globus pallidus internus), which ultimately manifests as an overwhelming inhibition of the key targets of the basal ganglia, such as the anterior thalamus and the brainstem structures controlling gait.7 Although the previous model made specific predictions regarding the likely behavioural predictors of freezing behaviour, the recent insights gained from the aforementioned neuroimaging and neurophysiological studies have helped to clarify the role of impaired corticostriatal coupling in the pathophysiological manifestation of FOG. For example, there is now evidence to suggest that impairments in neural coupling may precede freezing, providing a potential therapeutic avenue5.
In addition, the neuroimaging studies above have also provided clues that help to link the impaired corticostriatal coupling to the final manifestation of freezing, which is most likely related to increased firing within the output structures of the basal ganglia. These results implicate dysfunction within the ‘hyper-direct’ pathway of the basal ganglia, which links regions of the medial frontal cortex (such as the pre-supplementary motor area or pSMA) with the subthalamic nucleus of the basal ganglia.8 Through its connection to the pSMA, the STN is able to bypass the striatum and directly drive an increase in inhibitory GABAergic output from the output structures of the basal ganglia, such as the internal segment of the globus pallidus (GPi). Increased activity in the GPi, which is a member of the direct pathway of the basal ganglia, leads to an increase in the rate of inhibitory output onto the brainstem and thalamic structures that control the output of effective motor behaviours7 (see Figure 1). Importantly, the STN has been shown to increase its firing rate during periods of response conflict,9 suggesting that transient increases in STN firing may represent the pathophysiological link between impaired corticostriatal coupling during periods of increased response conflict, leading to an increase in the inhibitory output of the basal ganglia.7 This overwhelming inhibition of the brainstem structures controlling gait, such as the PPN, would impair motor initiation due to the PPNs efferent connectivity with the central pattern generators in the spinal cord controlling gait.10
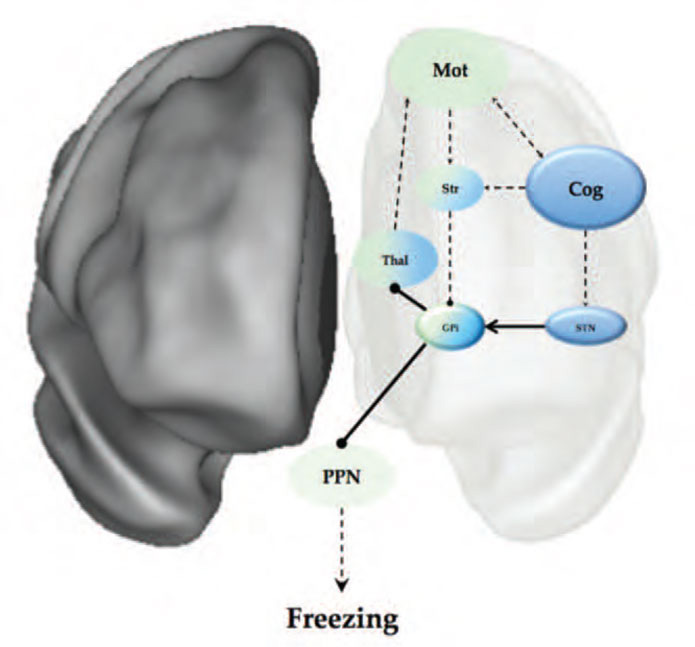
These proposed roles of the STN are well supported by both behavioural11 and neuroimaging evidence.12 For example, the STN has been consistently implicated in set-shifting behaviour,13 which has been shown to be impaired in patients with freezing.14 Furthermore, the role of the STN in freezing is aligned with the previously described functional neuroimaging studies that showed evidence that the globus pallidus and the STN enter into a low energy oscillatory state during freezing behaviour.4,5
These new insights into the pathophysiological mechanism of freezing behaviour suggest a number of exciting directions for future studies. For instance, the VR task could be combined with direct cellular recordings during deep brain stimulation surgery to test the hypothetical prediction that freezing is associated with increased firing within the STN. In addition, the combination of these insights with the temporal predictive capacity of EEG could also potentially inform future therapeutics, perhaps utilising specific neurophysiological signatures to ‘tune’ a closed loop deep brain stimulation feedback system15 that might even offer the potential of aborting freezing episodes.
References
- Giladi N, Kao R, Fahn S. Freezing phenomenon in patients with parkinsonian syndromes. Mov Disord. 1997;12(3):302-5.
- Naismith SL, Lewis SJG. A novel paradigm for modelling freezing of gait in Parkinson’s disease. J Clin Neurosci. 2010;17(8):984-7.
- Shine JM, Matar E, Bolitho SJ, Dilda V, Morris TR. Modeling freezing of gait in Parkinson’s disease with a virtual reality paradigm. Gait Posture. 2012;38(1):104-8.
- Shine JM, Matar E, Ward PB, Bolitho SJ, Gilat M, Pearson M, et al. Exploring the cortical and subcortical functional magnetic resonance imaging changes associated with freezing in Parkinson’s disease. Brain. 2013;136(Pt 4):1204-15.
- Shine JM, Matar E, Ward PB, Frank MJ, Moustafa AA, Pearson M, et al. Freezing of gait in Parkinson’s disease is associated with functional decoupling between the cognitive control network and the basal ganglia. Brain. EPub ahead of Print.
- Shine JM, Moustafa AA, Matar E, Frank MJ, Lewis SJG. (2013). The role of frontostriatal impairment in freezing of gait in Parkinson’s disease. Front Syst Neurosci. 7:61.
- Lewis SJG, Barker RA. A pathophysiological model of freezing of gait in Parkinson’s disease. Parkinsonism Relat Disord. 2009;15(5):333-8.
- Aron AR, Robbins TW, Poldrack RA. Inhibition and the right inferior frontal cortex. Trends Cogn Sci. 2004;8(4):170-7.
- Cavanagh JF, Wiecki TV, Cohen MX, Figueroa CM, Samanta J, Sherman SJ, et al. Subthalamic nucleus stimulation reverses mediofrontal influence over decision threshold. Nature Neurosci. 2011;14(11):1462-7.
- Lemon RN. Descending pathways in motor control. Annu Rev Neurosci. 2008;31:195-218.
- Frank MJ. Hold your horses: A dynamic computational role for the subthalamic nucleus in decision making. Neural Networks. 2006;19(8):1120-36.
- Aron AR, Behrens TE, Smith S, Frank MJ, Poldrack RA. Triangulating a Cognitive Control Network Using Diffusion-Weighted Magnetic Resonance Imaging (MRI) and Functional MRI. J Neurosci. 2007;27(14):3743-52.
- Block AE, Dhanji H, Thompson-Tardif SF, Floresco SB. Thalamic-prefrontal cortical-ventral striatal circuitry medicates dissociable components of strategy set shifting. Cereb Cortex. 2007;17(7):1625-36.
- Shine JM, Naismith SL, Palavra NC, Lewis SJG, Moore ST, Dilda V, et al. Attentional set-shifting deficits correlate with the severity of freezing of gait in Parkinson’s disease. Parkinsonism Relat Disord. 2012;19(3):388-90.
- Rosin B, Slovik M, Mitelman R, Rivlin-Etzion M, Haber SN, Israel Z, et al. Closed-loop deep brain stimulation is superior in ameliorating parkinsonism. Neuron. 2011;72(2):370-84.